
Most people, when imagining an atom, imagine a small nucleus of protons and neutrons, around which one or more electrons move. This view is based on a particle-based interpretation of quantum mechanics. But it is not enough to describe atoms under standard conditions.
If you want to reveal the secrets of the Universe, you only need to interrogate it until it gives answers in such a form in which you can understand them. When two quanta of energy interact - whether they are particles or antiparticles, massive or massless, fermions or bosons - its result, in principle, can tell you about the rules and laws that this system obeys. If we know about all possible variants of the results of any interaction, including their relative probabilities, only then can we say that we understand what is happening.
Surprisingly, everything we know about the universe can be tied in one way or another to the most humble entity we know of: the atom. An atom is the smallest unit of matter that still retains the unique characteristics of the macroscopic world, such as physical and chemical properties. And at the same time it is fundamentally a quantum entity, with its energy levels, properties and conservation laws. Moreover, this unremarkable atom is associated with all four known fundamental interactions. In a single atom, you can actually see all of physics. And here's what she can tell us about the universe.
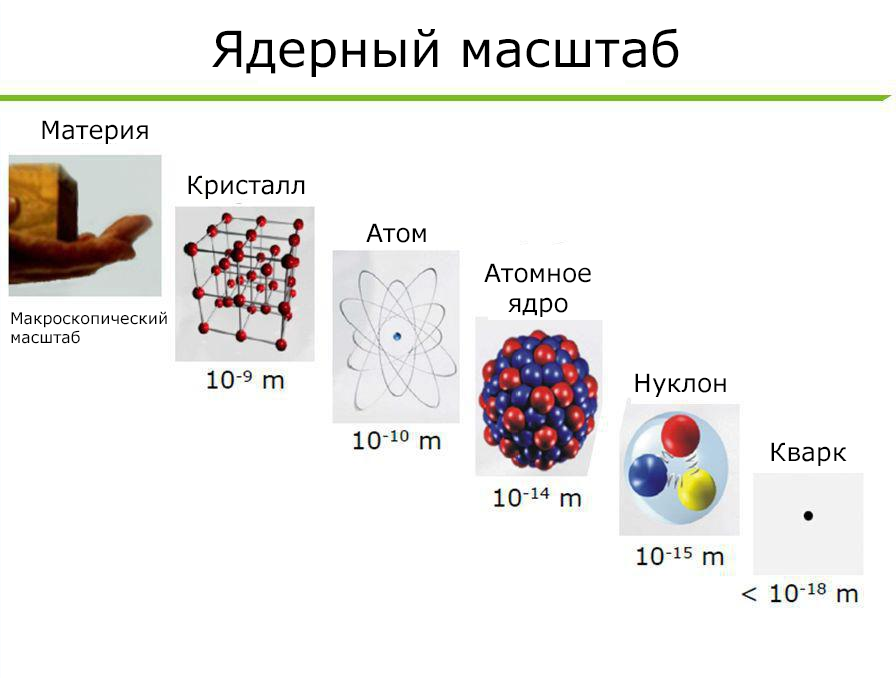
From macroscopic to subatomic scales, the size of fundamental particles plays a small role in determining the size of composite structures. It is not yet known if these building blocks are truly fundamental and pointlike particles, but we understand how the universe works from large, cosmic scales to small, subatomic ones. The human body contains about 10 28 atoms.
Here on Earth there are about 90 elements that appeared naturally - as a result of the course of the cosmic processes that created them. Essentially, an element is an atom whose nucleus is made up of protons, (and possibly) neutrons. There are several electrons around the nucleus, the number of which is equal to the number of protons. Each element has its own set of properties, including:
- hardness,
- Colour,
- melting and boiling point,
- density (amount of mass per volume),
- conductivity (how easy it is for electrons to move when an electrical voltage is applied),
- electronegativity (how tightly an atomic nucleus holds onto electrons when it is bonded to other atoms),
- ionization energy (how much energy is required to knock out an electron),
as well as many others. Interestingly, the type of atom (and, therefore, all of its properties) is determined by only one quantity: the number of protons in the nucleus.
The variety of atoms and the quantum rules governing electrons moving in orbit around nuclei - identical particles - make it possible without exaggeration to say that everything under the Sun consists of atoms - in one form or another.
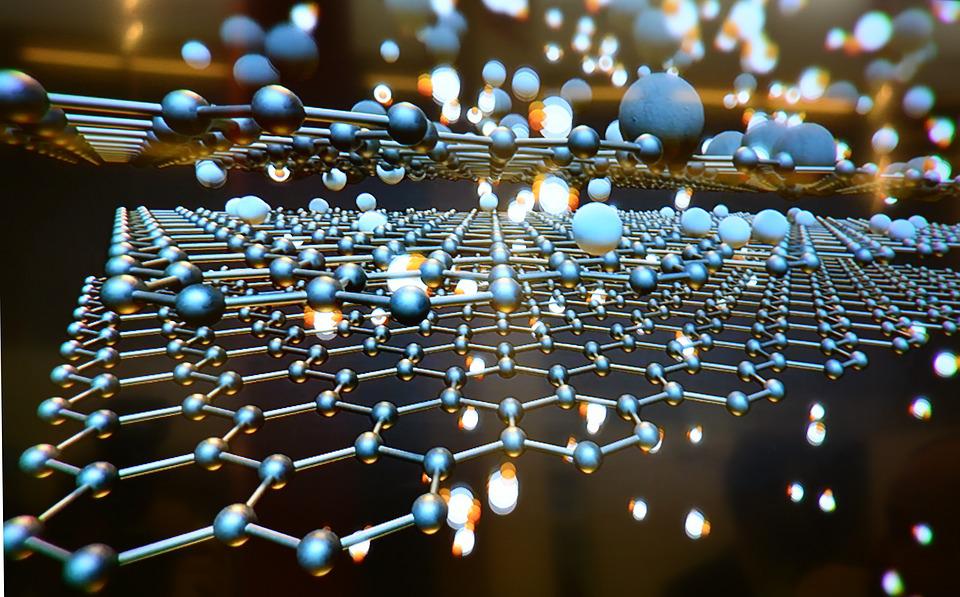
. , , . , – , , . , , .
Each atom with a unique set of protons in the nucleus forms unique bonds with other atoms, so there can be an almost infinite number of types of molecules, ions, salts and larger structures. Subatomic particles act on each other mainly with the help of electromagnetic forces. As a result, over time, macroscopic structures are formed, which we observe not only on Earth, but throughout the entire Universe.
A common property of all atoms is their mass. And the more protons and neutrons in the nucleus, the greater the mass of the atom. And although these are quantum entities, and the diameter of one atom does not exceed one angstrom, the range of action of gravity is not limited by anything. Any object with energy - including the rest energy that gives particles mass - will warp the fabric of spacetime according to Einstein's theory of general relativity. No matter how small the mass is, no matter how small the distance, the curvature of space-time caused by any number of atoms - be it 10 57 atoms in a star, 10 28 atoms in a human body, or one atom of helium - will occur exactly in accordance with General relativity.

The appearance of mass in an empty three-dimensional lattice causes its lines to bend in a certain way. They seem to be stretched towards the mass. The curvature of space due to the gravitational effect of the Earth is one of the ways to visualize gravity, and the fundamental difference between general and special relativity.
There are also electrically charged particles in atoms. Protons have a positive electrical charge; neutrons are neutral; the charge of electrons is equal in magnitude and opposite in sign to the charge of protons. All protons with neutrons are bound in an atomic nucleus with a diameter of only 10 -15m (femtometer), and electrons are in a cloud 100,000 times larger. Each electron is at its own unique energy level, and can move from level to level only with a discrete change in energy.
This is remarkable for two reasons. First, when one atom approaches another (or a group of atoms), they can interact. At the quantum level, their wave functions can overlap, and atoms bind together to form molecules, ions, and salts. These connected structures have their own unique shapes and configurations of electron clouds. Accordingly, they have their own unique energy levels, absorbing and emitting photons only at certain wavelengths.
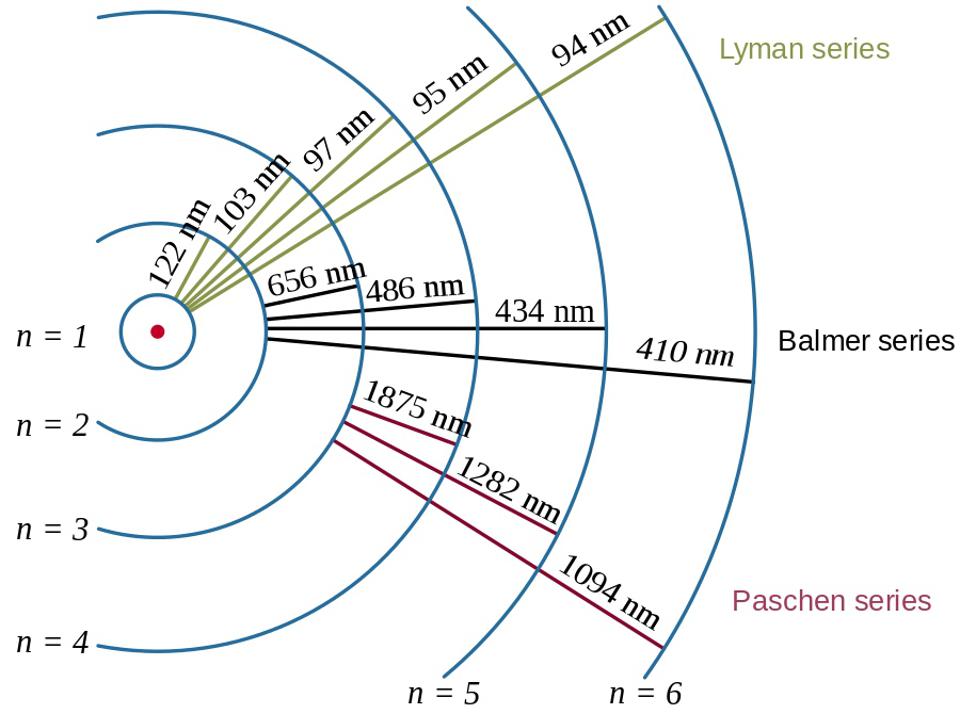
Atomic electronic transitions in the hydrogen atom and the wavelengths of the resulting photons demonstrate the bond energy and the relationship between an electron and a proton in quantum physics.
These electronic transitions in an atom or a group of atoms are unique: they are different for each atom or configuration from a group of atoms. By detecting the spectral lines of an atom or molecule - it does not matter whether it is absorption or emission - you can immediately tell what kind of atom or molecule it is. Internal electronic transitions are consistent with a unique set of energy levels, and electron transitions unambiguously indicate the type and configuration of the atom / atoms.
Throughout the universe, atoms and molecules obey the same rules: the laws of classical and quantum electrodynamics, which govern all charged particles. Even inside the atomic nucleus itself, consisting of charged quarks and gluons without an electric charge, electromagnetic connections play a critical role. This internal structure explains why the magnetic moment of a proton is almost three times stronger than the magnetic moment of an electron (and has the opposite sign), while a neutron has a magnetic moment that is almost twice that of an electron and has the same sign.
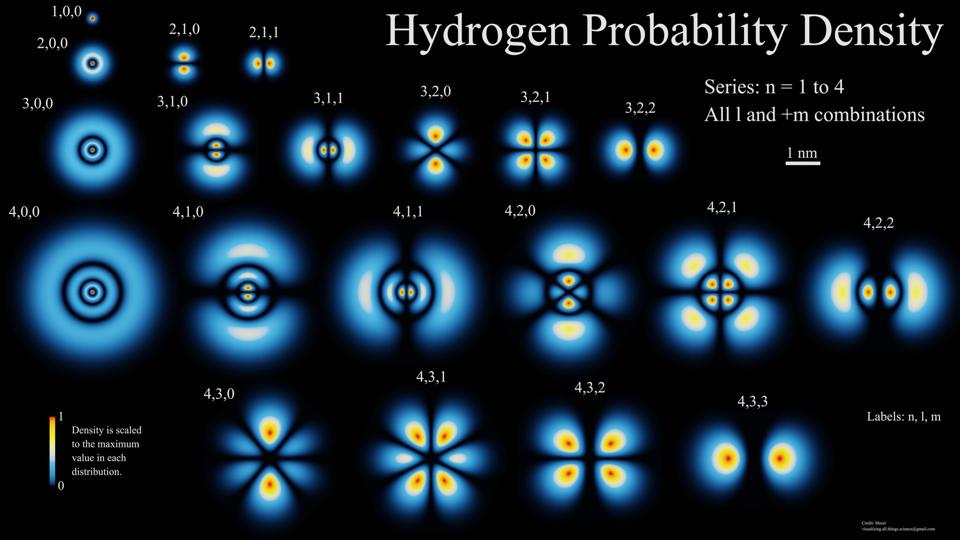
The lowest energy level of hydrogen (1S) at the top left has a very dense probabilistic electron cloud. At higher energy levels, the clouds are similar, but have a more complex structure. For the first excited state, there are two independent configurations: 2S and 2P, which, due to an almost imperceptible effect, produce different energy levels.
Although electrical interaction works over fairly long distances - in fact, like gravity, it also has no limitations - the electrical neutrality of the atom as a whole plays an incredibly important role in understanding the behavior of the entire universe. Electromagnetic interaction is incredibly strong - two protons are repelled with a force 10 36 times greater than their gravitational attraction!
But since the macroscopic objects we are accustomed to consist of so many atoms, and since the atoms themselves are electrically neutral, we notice something only if:
- the object has an electric charge, like a charged electroscope,
- when the charge flows from place to place, as in a lightning strike,
- when the charges separate, creating an electrical potential, like in a battery.
One of the simplest and most interesting examples of these processes can be seen by rubbing a balloon on your own T-shirt, and then trying to lean it against your hair or a wall. The ball will stick because the transition or redistribution of a small number of electrons gives the object a charge that can overcome the force of gravity. These are van der Waals forces , they act between molecules, and even objects in general neutral can have an electromagnetic interaction that can resist gravity at short distances.
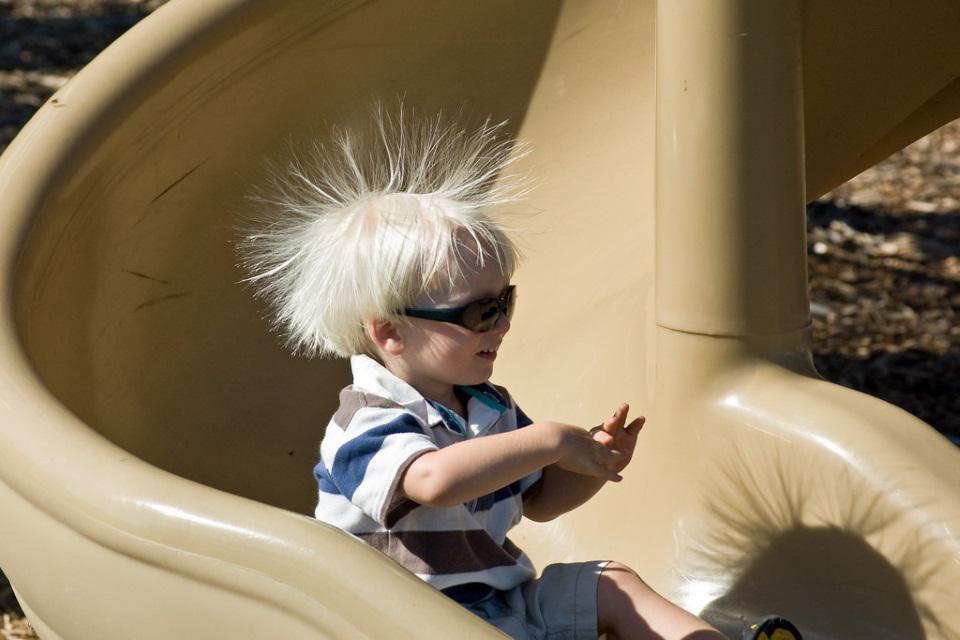
By rubbing two different materials, like cloth and plastic, you can transfer charges from one to the other, causing both objects to be charged.
At the classical and quantum levels, a huge amount of information associated with electromagnetic interactions is encoded in the atom, while the "classical" (not quantum) general relativity is sufficient to explain the atomic and subatomic interactions we have ever encountered. If you get even deeper into the atom, inside the protons and neutrons, you can reveal the nature and properties of the remaining fundamental interactions: weak and strong.
As you go down to the femtometer scale, you first begin to notice the effects of strong interactions. For the first time, it manifests itself between different nucleons - protons and neutrons, of which any nucleus consists. The electrical interaction between two nucleons either repels them (the charges of the protons are the same), or does not arise (the neutrons have no charges). But at small distances, there is an interaction that is even stronger than electromagnetic: a strong interaction that works between quarks through the exchange of gluons. Different protons and neutrons can exchange pairs of quarks-antiquarks - mesons - this binds them in the nucleus, and with a suitable configuration overcomes the force of electromagnetic repulsion.
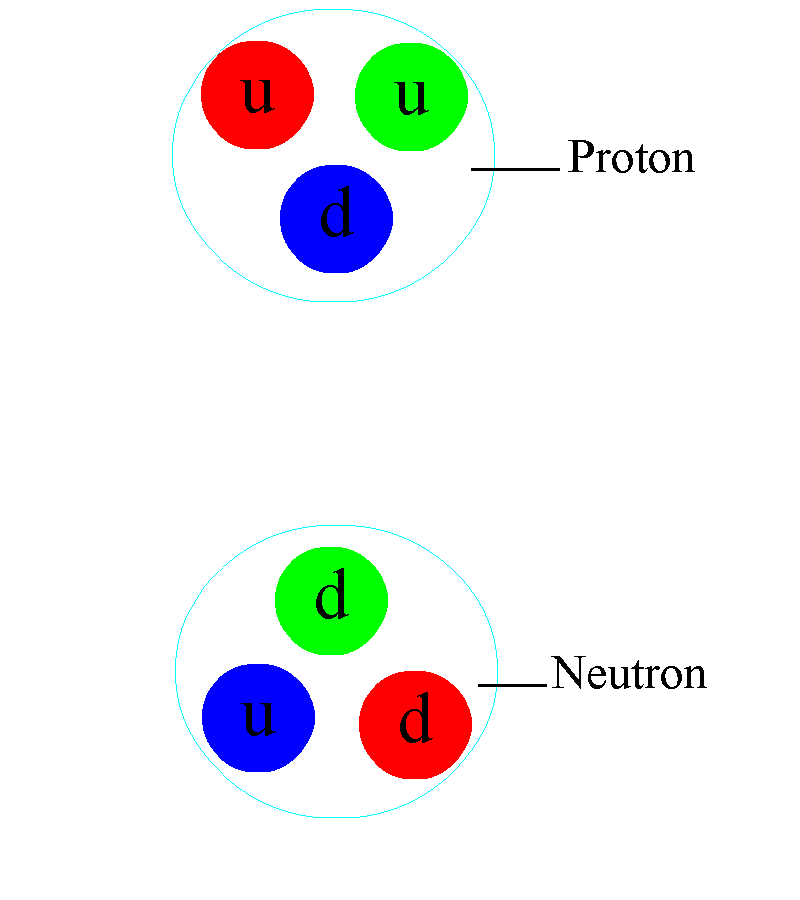
«», – . , , .
In the depths of the atomic nucleus, the strong interaction manifests itself in a different way: individual quarks are constantly exchanging gluons. In addition to the gravitational charge (mass) and the electromagnetic charge inherent in matter, there is also a charge characteristic of quarks and gluons: colored. They don't just always attract, like gravity, or they have two charges that can be repelled or attracted like electric ones. They have three independent colors — red, green, and blue — and three anti-colors. They are found only in a “colorless” combination, in which all three colors (or anti-colors) are combined, or color-anti-color combinations are combined.
Keeping protons and neutrons intact is the exchange of gluons - especially when the quarks move away and the strong force increases. The more energy you can transfer through collisions with subatomic particles, the more quarks, antiquarks, and gluons you can see. It looks like the proton's insides are filled with a whole sea of particles, and the harder you hit it, the more sticky they become. Going deeper by the amount of the maximum energy available to us, we still do not see any restrictions on the density of these subatomic particles inside atomic nuclei.
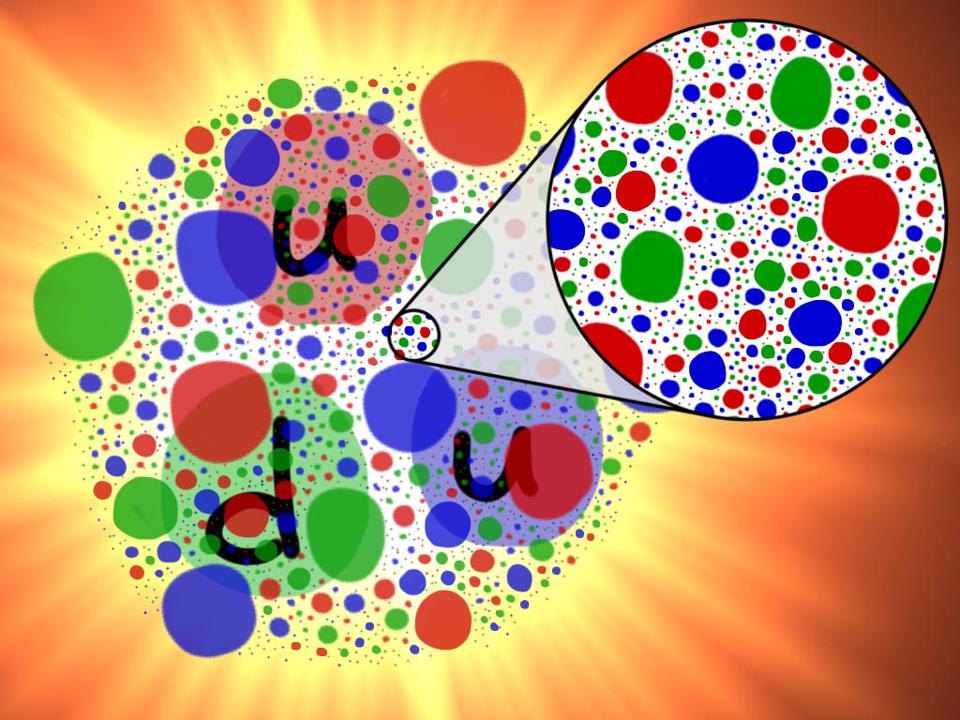
The proton is not just three quarks with gluons. It is a whole sea of dense particles and antiparticles. The more we study the proton, the more energy we spend on inelastic collisions, the more internal structure we find in it.
But not every atom is able to live forever in a stable configuration. Many atoms undergo radioactive decay - sooner or later they emit one or more particles, which fundamentally changes their properties. The most common type of decay is alpha decay , in which an unstable atom spits out a helium nucleus with two protons and two neutrons. The second most common type is beta decay , in which an atom spits out an electron and an anti-electron neutrino, and one of the neutrons in the nucleus turns into a proton.
This requires another new force: the weak nuclear force. It depends on its type of charge - weak, which is a combination of weak hypercharge and weak isospin . Weak charges have proven to be extremely difficult to measure, since weak interactions are millions of times weaker than strong or electromagnetic - until you go down to extremely small scales, such as 0.1% of the diameter of a proton. Weak interactions can be observed in a suitable atom, ready for beta decay. It turns out that all four fundamental interactions can be probed simply by studying the atom.

Schematic representation of the beta decay of a massive atomic nucleus. The neutron turns into a proton, an electron and an anti-electron neutrino. Before the discovery of neutrinos, it seemed that energy and momentum were not conserved in beta decays.
Something remarkable follows from this: if you take any particle of the Universe, even not yet open, but subject to one of these interactions, it will also interact with atoms. Through interactions with particles inside an unremarkable atom, we have discovered a huge number of particles - including all kinds of neutrinos and antineutrinos. An atom is both what we are made of and a window into the true nature of matter.
The deeper we look into the building blocks of matter, the better we understand the nature of the universe itself. Only by interrogating the Universe on the subject of what rules all particles and antiparticles obey, and how they bind to each other, can we understand its structure. And while science and technology, subject to us, allow us to dig deeper and deeper, it would be a shame to refuse research only because they cannot guarantee us some new revolutionary discovery. The only thing that is known for sure: if we do not dig deeper, we will never find anything.