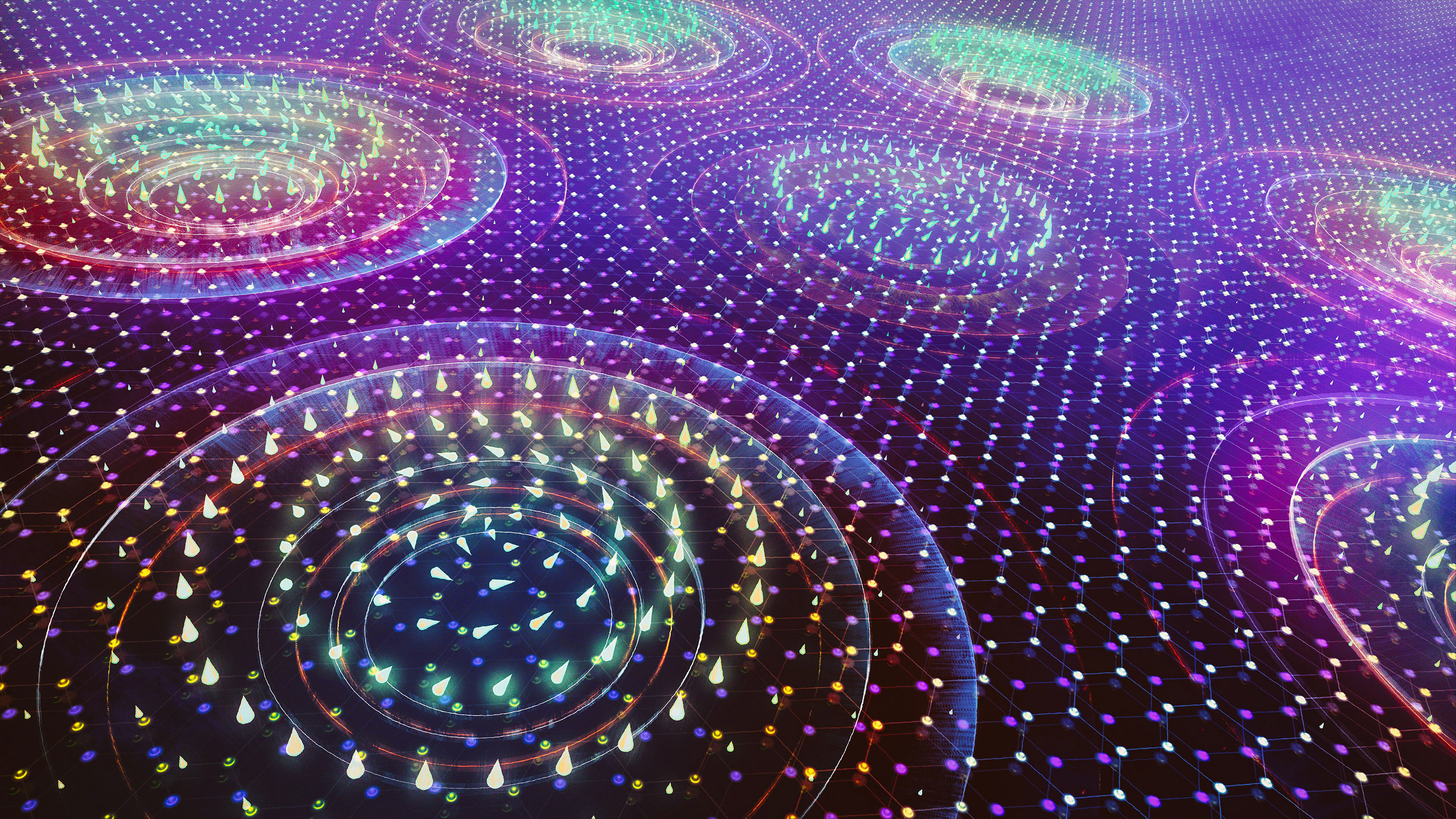
Skyrmions arise from the collective behavior of many electrons, but behave like individual particles.
For the past three years electrons have been playing games for physicists.
The game began in 2018 when Pablo Jarillo-Herrero's lab announced a find of a decade : when researchers stacked one layer of carbon atoms on top of another, applied a “magic” twist of 1.1 degrees between them, and then cooled the atomic plates to near absolute zero. then the sample became an ideal conductor of electrons.
How did the particles conspire to glide flawlessly through graphene sheets? The kaleidoscopic "moire" created by the tilt angle seemed like a significant result, but no one was sure. To find out, the researchers began folding and twisting (turning) any material they could get their hands on.
At first, the electrons played along. A series of experiments have shown that in many flat materials, low temperatures cause a sharp drop in electrical resistance. It seemed that the conditions necessary for ideal conductivity were already better understood, and, therefore, that alluring step towards the revolution in electronics was close.
"It felt like superconductivity was just everywhere," said Matthew Jankowitz , a condensed matter physicist at the University of Washington, "no matter which system you looked at."
But the electrons suddenly "put on a mask of false modesty." As the researchers studied the samples more closely, the cases of superconductivity disappeared. In some materials, the resistance did not actually drop to zero. There were conflicting results in the various samples studied. Only in the original bilayer graphene did electrons actually move without "resistance" in most cases.
"We had a whole zoo of different twisted materials, and twisted two-layer graphene was the only superconductor," Jankowitz said.
Then, over the past month in two articles published in the journals "Nature" and "Science", another superconductor was described, a three-layer graphene "sandwich" with two even outer, "bread" sheets and a filling sheet rotated by 1.56 degrees.
The unmistakable ability to transfer electrons of twisted three-layer graphene confirms that the two-plate system was not an accident. "It was the first of a family of moire superconductors," said Jarillo-Herrero, a physicist at the Massachusetts Institute of Technology who also led one of the new experiments, and this is the second member of the family.

Samuel Velasco / Quanta Magazine; Source: Courtesy of Pablo Jarillo-Herrero
Image comment
-
, . , 1,56 .
, . , 1,56 .
Importantly, this second "brother" helped shed light on the underlying mechanism that could be responsible for the superconductivity of these materials.
A few months after the 2018 discovery, one group of theorists began to puzzle over the mechanism that made bilayer graphene a superconductor. They suspected that one particular geometric feature could allow electrons to swirl into exotic vortexes that behave in completely new ways. This mechanism, which is unlike any of the (few) known superconducting circuits, could explain the success of bilayer graphene superconductivity, as well as the failures of other materials. He also predicted that graphene's three-layer "brother" would also be a superconductor.
But this remained just a theory, at least until the laboratories were able to test it. “From what we know now, this direction seems exciting,” said Eslam Khalaf , a Harvard University researcher who helped develop the model. "It's not every day that a new way of producing superconductivity appears."
Three miracles
In a chaotic world where friction abounds and particles never remain stationary, such a perfect phenomenon as superconductivity has no right to exist. However, common metals such as mercury regularly show themselves at low temperatures, as Heike Kamerlingh Onnes accidentally discovered in the early 20th century.
The secret is that near absolute zero vibrations in the atomic lattice of a metal break free electrons into pairs. These pairs interact in a way that individual electrons cannot interact, forming a single quantum mechanical "superfluid" that flows through the material without a single collision of electrons with an atom (which generate heat and resistance). The original theory of superconductivity, developed back in 1957, described it as a sophisticated electronic "dance" that can be disrupted by all but the most ideal environments. “It's kind of a miracle that they do connect at all, because electrons repel each other so much,” said Ashwin Vishwanath , a theoretical physicist at Harvard.
In 1986, researchers noticed electrons performing a second miracle, this time in a family of copper compounds known as cuprates. The materials could somehow maintain superconductivity tens of degrees above the temperature that often separates ordinary electron pairs. There seemed to be a new mechanism at work, which is probably related mainly to the electrons themselves, and not to their atomic framework.

Ashwin Vishwanath's team came up with a way to understand superconductivity in graphene by studying its geometric structure. Courtesy of Ashwin Vishwanath
But after decades of intense studyResearchers are still not sure exactly how the electrons in cuprates govern their superconducting abilities. Predicting the behavior of electronic conglomerates involves calculating the brute force of each particle on each other - a calculation that grows in complexity exponentially as the number of electrons increases. To understand even the smallest particle of a superconductor, theorists need to understand the behavior of the trillions of electrons. The current simulation can handle about a dozen.
Experimenters are not in the best position right now. They can grow new crystals by exchanging one atom for another and test their properties. But the material doesn't reveal what the electrons are doing inside. And the researchers don't know how the material will behave until they make it. “Nobody could tell that I was going to make this new [cuprate],” said Yankowitz, “and predict what [the temperature at which it becomes a superconductor] would be. Now it is a terribly difficult task. "
The unique properties of twisted bilayer graphene made it more transparent than cuprates. Instead of creating a completely new substance, experimenters could change the properties of graphene with just an electric field, which made it, according to many researchers, a "playground" for superconductivity.
“This is an exciting challenge and a remarkable feature of twisted bilayer graphene,” said Subir Sachdev , condensed matter physicist at Harvard. "This provides a whole new set of tools for studying the motion of electrons."
He also offered theoretical guidance. At a magic angle of 1.1 degrees, graphene's honeycomb lattices are connected in such a way that usually fast electrons move slowly - physicists describe this material as "flat stripes." Inert electrons spend more time together, which gives them the opportunity to organize.
But the leadership was vague. Electrons in materials with flat stripes can communicate in many ways, and superconducting pairing is just one of them. Researchers stacked many atomic plates at magic angles to smooth out the stripes, but the superconducting lightning didn't want to be caught in the bottle.
They seemed to be missing something important.
Vortex skyrmions
In March 2018, shortly after the discovery of superconductivity in twisted graphene, Vishwanath and his colleagues attempted to demystify the magic angle and understand what could hold electrons together.
It was impossible to write a theory that would completely reflect the movement of rebellious electrons in bilayer graphene, so theorists started by imagining particles that behaved slightly better. They viewed the hexagonal graphene lattice as two sublattices of triangles. When electrons move from atom to atom, they usually "jump" to an atom on the opposite grid. Sometimes a rebel jumps to an atom in the same grid.

Image comment
. , .
. , .
Vishwanath and company insisted that electrons were always changing the grid. This choice made it mathematically cleaner to subdivide the hexagonal grid into triangular ones. And in two-layer graphene, with its two layers, one obscure feature was discovered that eventually became important: the electrons, being confined in this way, began to move as if they were under the influence of a magnetic field. In particular, electrons in one sublattice apparently felt a positive magnetic field, while electrons in the other sublattice felt negative. Theorists weren't quite aware of this, but the key to a new theory of superconductivity lay right on the surface.
When the theory was applied to get the magic angle of 1.1 degrees in August 2018 in bilayer graphene, Vishwanath and colleagues began to build up the number of graphene layers. The theory, which was originally developed for two layers, applied to the new structures much better than expected. They found they could calculate the magic angle for each successive graphene stack using simple relationships that seemed out of reach for the increasing complexity of more massive systems.
“In condensed matter physics, you especially notice that you are doing something very close to physical or even practical reality, but from time to time you see this most ideal world, which is hidden behind,” said Vishwanath.
As the group carried out further research, adding more realistic details to the theory, superconductivity emerged, but in a completely new way. It is possible that not pairs of electrons were formed, but streams of electrons known as skyrmions... Since bilayer graphene consists of two layers, it has four sublattices, but these sublattices with the same magnetic charge act as one. Effective magnetic fields cause electrons visiting atoms in one grid to tend to roughen the surface, while electrons on another grid tend to make it smooth. This configuration can lock electrons in place so that the system behaves like an insulator. (Interestingly, experiments with cuprates and twisted bilayer graphene suggest that both materials act as insulators just before they become superconductors.)
But if you disturb the balance with an additional charge, the electrons on each sublattice can take on a collective vortex pattern - a skyrmion - where a spinning electron at the epicenter of a storm roughens (or smoothes it), and its neighbors are smoothed out in a spiral manner.

Image comment
— , . , , , .
— , . , , , .
Although thousands of electrons can enter the skyrmion of graphene, the vortex acts as if it were one particle with the charge of one electron. You might expect negative skyrmions to repel each other, but the quantum mechanical rules governing how electrons "jump" between two sublattices actually attract skyrmions on opposite grids together. In other words, they form pairs of electron-like charges - a fundamental requirement for superconductivity.
The key to the skyrmion story is the 180-degree rotational symmetry, which determines the transfer of electrons between triangular sublattices. The rectangle has the same symmetry. Both the hexagon and the rectangular or hexagonal lattice have it. But folding and twisting sheets of anything other than graphene breaks that order. Finally, Viswanath and his colleagues were able to explain why the twisted lattice zoo failed to become a superconductor.
“That was the moment when it all came together,” Khalaf said.
Theory and graphene
Jarillo-Herrero already thought that something good can come out of three layers. Electrons in materials with flat stripes move slowly enough for the particles to work together, but superconductivity can be enhanced by "dispersing" stripes, through which vapors move more easily. For twisted two-layer graphene, the former is characteristic. The last statement is typical for single-layer graphene. Putting them together can give us the best of both worlds.
Then came the prediction of the Vishwanath group that 1.5 degrees is the magic angle for creating superconducting skyrmions in three layers of graphene.
Keeping these arguments in mind, Jarillo-Herrero's laboratory as well as Philip Kim's laboratory at Harvard began to create three-layer stacks of graphene sheets. Both laboratories saw everything the theorists predicted and more.

Pablo Jarillo-Herrero

Laboratory Philip Kim Laboratory.
Photo source: Bryce Wickmark; Eliza Grinnell / Harvard SEAS
If two-layer graphene is a platform for superconductivity, then three-layer graphene is then a real celebration and festival. Experimenters can not only fine tune the number of electrons in the lattices, they can also arbitrarily move electrons between layers using a second electric field. With this flexibility, researchers can search for superconducting sweet spots, making electrons feel like they are moving through a two-layer system, a single-layer system, or any number of hybrid systems.
Using this unprecedented customization capability, labs have confirmed that, unlike other twisted materials, three-layer graphene passes all superconductivity tests. They also found several indirect indications that superconductivity occurs in unusual ways.
First, electrons interact very well. In conventional superconductors, where clusters of atoms pair up free electrons, only 1 electron in 100,000 joins the superconducting superfluid. Cuprates involve about 1 in 30 free electrons. But in the three-layer system, according to researchers, every tenth person participates.
The elements in superconducting pairs - whether they are electrons or skyrmions - are also quite close together. The ends of electron pairs in supercooled aluminum are spaced 10,000 times the average distance between electrons, which is like a soup of long spaghetti. And in three-layer graphene, superconducting pairs bunch together like macaroni, and the elements are equally close to both the “partner” and the “neighbors”.
Given how difficult it is to know everything that happens inside a material at the subatomic level, it is too early to say that skyrmions provide superconductivity in multilayer graphene. But for Halaf, the strange behavior that Jarillo-Herrero and Kim have observed converges with electron vortices.
Unlike standard electron pairs, skyrmion pairs bond tightly to produce highly efficient superconductivity. Composite objects are also large and closely spaced.
And in standard metals, electrons, falling into a state that presupposes a choice from a variety of possible actions, lead to strong superconductivity. But when the researchers gave that kind of freedom to electrons in a three-layer system, superconductivity disappeared. According to Khalaf, this may be because the increased freedom allows skyrmions to fall apart.
“I don’t think we can definitely consider this superconductor unconventional,” said Corey Dean., a condensed matter physicist from Columbia University. But he added that the unusual reaction to the increased freedom "definitely points in the opposite direction."
If the rotational symmetry that Vishwanath and his colleagues have identified is indeed critical to the superconductivity of multilayer graphene, materials scientists may one day be able to use this fact to orient themselves in a field of many billions of possible materials and find a lattice that can hold electrons together on a warm day.
The charges in twisted graphene are too thinly distributed across giant moire cells for superconductivity at high temperatures, but the bond holding them together - whether skyrmions or whatever - seems strong. The researchers hope that further study of twisted graphene and theories explaining its unusual properties will explain its reliable superconductivity and point the way to a lattice that can absorb more heat.
“If you get the same effect on the scale of atoms, then it will really apply,” Sachdev said. (I omitted it, summarized it)