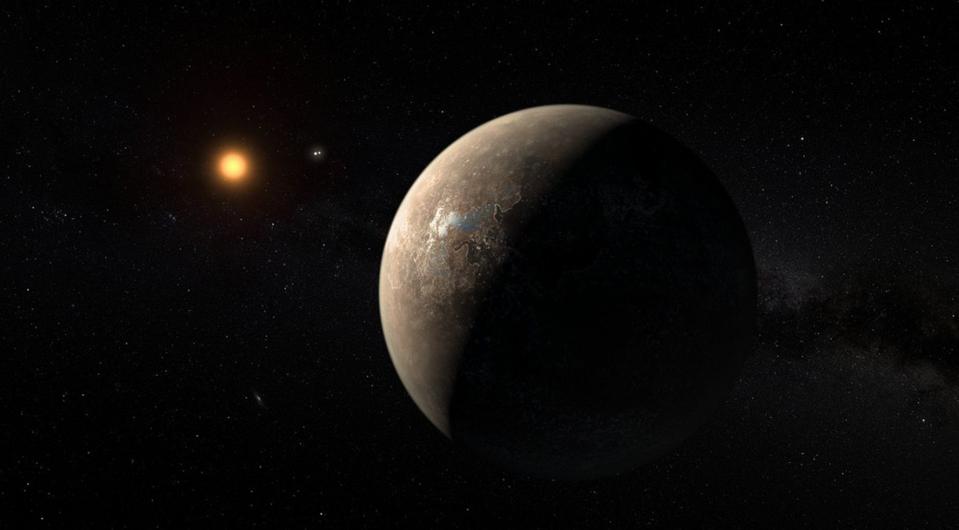
How an artist sees the exoplanet Proxima b . It is believed that it is unfriendly for life due to the fact that it does not have an atmosphere due to the properties of its parent star. This is, as astronomers say, the "gazing" world - one side of the planet is constantly looking at the star, and fries in its light, and the other freezes. Perhaps these are the planets most in the Universe.
There is one popular myth in astronomy that the sun is a typical star. If we are talking about the fact that the Sun does not stand out in anything special - then yes, it is. It consists of the same ingredients as the rest of the stars. It is 70% hydrogen, 28% helium, 1-2% other elements. It receives energy from nuclear fusion that occurs in the nucleus. In a sense, this is a "typical" star, included in the vast majority of about 10 24stars contained within the observable universe.
However, in reality, the Sun is brighter and more massive, and its lifespan is shorter than that of 95% of the stars in the Universe. If you choose any random star, then with a probability of 80% it will be a red dwarf - it will be smaller, colder, dimmer and less in mass than our Sun. Most stars are not like our Sun.
What about the planets? If we take into account only those that we have found to date - and this is already more than 4000 - we can conclude that most often there are planets slightly larger than the Earth. However, this is most likely not the case. If you're not careful, the universe can easily trick us - however, we have enough information to avoid it. And this is how we know about what type of planets in the Universe the most.
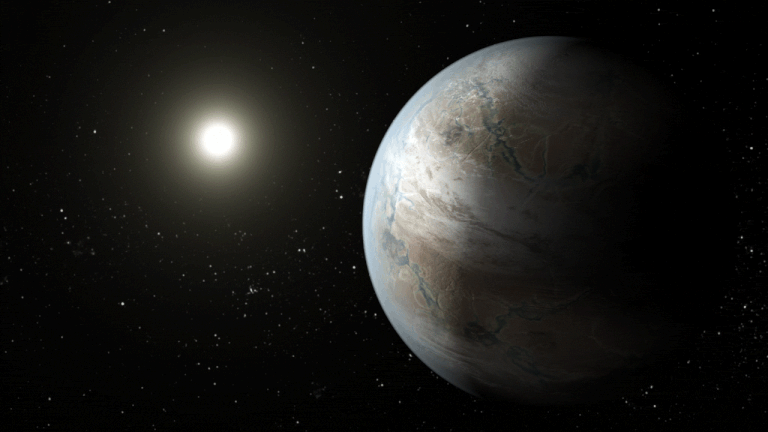
, , , . , . , .
When we first started studying exoplanets, the first planets found outside our solar system were unlike anything we've seen before. The first batch of such planets was found in the 1990s. They were exceptionally large and massive planets, huge even in comparison with Jupiter - the most massive planet in our solar system. In addition, they were not located as far from their star as our gas giants - they were extremely close, and it took them only a few days to complete a revolution around the star. The first planets like these found orbited even faster than Mercury, our innermost planet.
Did these so-called. "Hot Jupiters" to the most common type of planets? Not at all. But there was something special about them: it was to such planets that our first methods of detecting them were adapted. The very first of the successful techniques for detecting planets outside the solar system, we called the "stellar tremor" method: since a star gravitationally attracts a planet revolving around it, the planet in turn attracts it, with equal and opposite force. In fact, the planets do not move in an ellipse around their parent stars: both members of the planet-star system revolve around a common center of mass.

The radial velocity method, also known as the stellar tremor method for searching for exoplanets, is based on measuring the motion of a parent star under the gravitational influence of planets revolving around it. Since the planet and the star revolve around a common center of mass, the star does not stand still, but "trembles". Periodic red and blue shifts of the star's light make it possible to calculate the mass and orbital period of an exoplanet.
These stars are too far away and move too little laterally (side to side) for us to detect this movement. But movement in the radial direction, along the line of sight, can be detected. The properties of the light emanating from the star depend on its motion.
As a star moves towards us, the wavelength of its light shifts to higher frequencies, shorter wavelengths, higher energies, and blues. As a star moves away from us, the wavelength of its light shifts to lower frequencies, longer wavelengths, lower energies, and reds.
If you observe a star around which a massive companion planet revolves for a long time, you will periodically see how the star moves in your direction, then away from you, then back to you, etc. If it has several planets, then several signals will be superimposed. The original term, "stellar jitter", has gone out of fashion and we now call it the "radial velocity" method. We began to find planets only when our spectroscopic capabilities became sufficiently accurate. We split light into different wavelengths to look for specific elements as well as absorption and emission features.

Spectrum echelle (stepped grating) - this is how light was displayed on the Hamilton spectrograph in the 1990s. The system made it possible to measure the radial speed with an accuracy of 15-20 m / s - much more accurate than the previous methods. At that time, thanks to this breakthrough, several exoplanets were discovered, incl. and hot Jupiters.
And this is the first lesson in statistics. We found these "hot Jupiters" not because they are the most common type of planet in the universe. We found them because planets of this type were easiest to detect with a specific method. When using the radial velocity method, you need to ask yourself the question - what type of system will give the most visible effect? It turns out that in this case, three factors play the greatest role.
- , . , , 100 , , , 2 . , , , - , .
- , . 100 , , 100 .
- , , . , , , – , , , – . , .
Therefore, this method tends to find the planets closest to the star with a large mass and an orbit located edge-on to us. Not surprisingly, most of the first discovered planets turned out to be "hot Jupiters."

Field of view of the first search for the Kepler telescope against the background of the Milky Way (yellow cone). Most of the observations Kepler continuously observed the same part of the sky, simultaneously studying 100,000 stars. During the transit of the planet across the disk of the star, "Kepler" observed the periodic decay of its light.
Of course, the modern exoplanetary revolution began as soon as the Kepler telescope got involved and began collecting data. Instead of radial velocity, the main method for finding planets was made a highly sensitive transit method . Some of the systems whose planetary orbits are edge-to-edge are so well aligned that their planets pass right between us and their star. At these moments, they block out a small percentage of the star's light.
With an ideal orbital position, the star's brightness will fade regularly, since the star usually emits relatively evenly, but when a colder planet passes in front of it, some of the light will be blocked.
The scheme of Kepler's work was ingenious: the telescope looked at that place in the sky where a large stellar field is located, stretching along the nearest thickening of the spiral arm of the Galaxy. And in a region several thousand light years across, it was able to simultaneously observe over 100,000 stars, tracking regular decreases and variations in brightness. Although there are over 4,000 confirmed exoplanets known today (more than half of which have been found by Kepler), the discovery of a Mercury-like planet orbiting a star similar to our Sun is beyond our current technology. From Kepler's point of view, Mercury would be 285 times smaller than the Sun, which would make it even more difficult to notice than from Earth - hence its apparent size is 1/194 of the Sun.
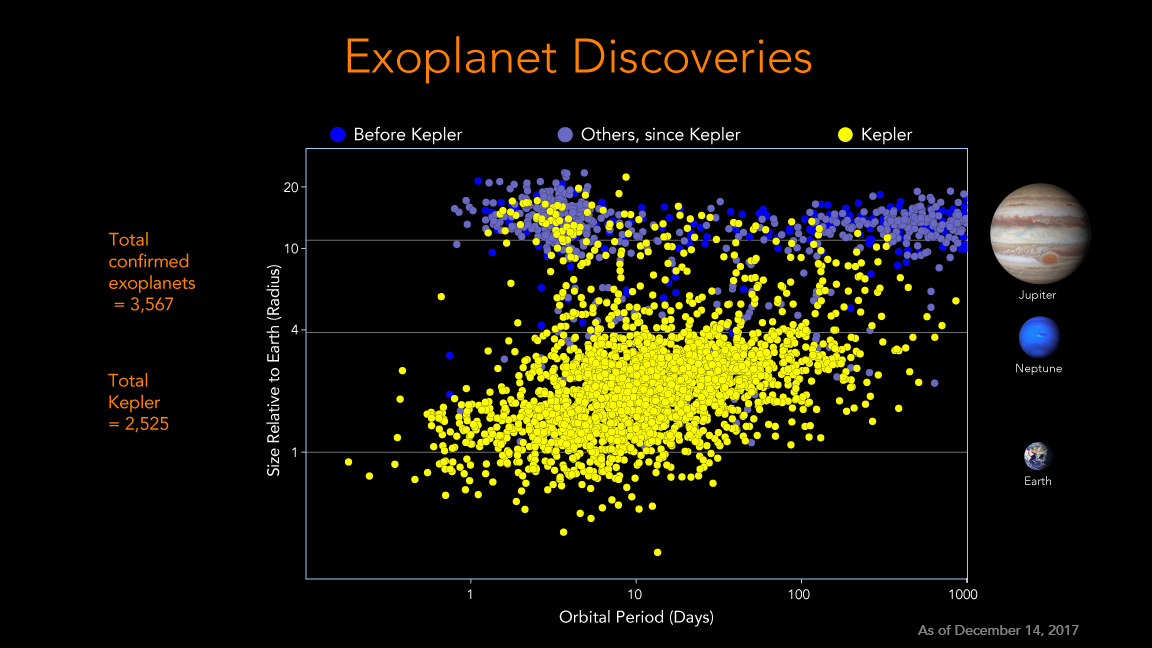
After Kepler did his job, we increased our collection of known exoplanets from a little over 100 to over 4,000. Most of the time Kepler observed the same 100,000 stars for three years, and found planets as large as Jupiter. and less than Earth. On the graph of the planets he discovered, it can be seen that the peak of detections is in the interval that belongs to the so-called. "Super-Earths". But the more we learn about exoplanets, the more likely it is that they will not actually be super-Earths, but mini-Neptunes - planets with a large content of unstable gas.
It is very tempting to conclude that the most common type of planet in the universe would be a super-Earth. Of course, after Kepler gave us planetary candidates, we confirmed their existence by measuring the radial velocity. But since Kepler told us where, when and how accurately to observe the star, we have the opportunity to check all the candidates he found. From the data obtained, it would be possible to conclude that the most common type of planets in the Universe will not be "hot Jupiter", but super-Earths. On the graph: the ratio of the radius of the planets to the brightness of the star. To the left are bright stars, to the right are dim ones. Higher on the graph are the larger planets. Orange - Kepler candidates. Blue - planets discovered by the transit method from Earth.

Most of the planets discovered by Kepler turn out to be larger than the Earth, and they revolve around dimmer stars than ours. However, large planets are rare in faint stars.
However, this conclusion will most likely be incorrect. While not prone to radial error, the Kepler mission in particular and the transit method in general have their own biases that fundamentally limit its capabilities. Imagine that we are looking at the solar system from afar. What are the chances that any planet will be so well oriented that it will pass directly in front of the Sun? And what is the most likely configuration?
The first distortion is simple: the closer a planet is to a star, the more likely it will pass in front of it. Inner planets, even with strongly inclined orbits, can still pass through the disk of a star - and in order for the outer planets to pass through the disk, their orbit must be very precisely aligned.

Orbits of the planets of the solar system, top view. It can be seen how precisely the orbital plane must be aligned for the planet to traverse the star's disk. In the case of Mercury, a slight tilt will still allow it to traverse the sun's disk. But the farther from the star, the more accurate the position of the orbit should be.
For a star the size of the Sun, the orbit of a planet the size of Mercury's orbit can deviate 1.37 ° from its ideal edge-on position, and Mercury can still traverse the star's disk with a 0.76% probability. The orbit of the same planet, located at a distance from the star comparable to the distance from the Sun to the Earth, can deviate by no more than 0.53 °, and the chances of its passage across the disk are 0.30%. At a distance, as from the Sun to Jupiter, the deflection drops to a magnitude of 0.101 °, and the probability of passing through the disk is 0.056%. In the case of Neptune, this will already be 0.0177 ° and 0.0098%.
Therefore, we should expect more frequent detection of planets located close to the star, and the further the planet is from the star, the more difficult it will be to find it. Over a three-year observation period, the vast majority of the planets discovered will rotate in closer orbits, and move along them faster than the planets in our solar system.

The main transit of the exoplanet along the star KOI-64 (L) and the departure of the planet behind the parent star ®. The first dip in brightness allows the transit to be roughly determined, and the additional information helps scientists know the various properties of the planet, apart from the radius and orbital period. Note that detecting a planet requires a signal no weaker than 100 ppm of the baseline (ppm).
And then there's the problem of physical size. A planet needs to block out much of the star's light to get into the Kepler dataset. And here a small compromise arises: a smaller planet passing through the disk of its star 30 times can block only one tenth of the light (which makes it about 3.2 times smaller) compared to a planet that passes through the disk of the star only 3 times ...
It turns out that we have two distortions working in pairs: we tend to detect planets located closer to the parent stars, because the chances of the correct location of their orbit in relation to us are higher, as well as planets whose sizes are larger in comparison with their parent stars. Therefore, analyzing the data from Kepler, we find that the distribution of the planets will be different for stars of different types.
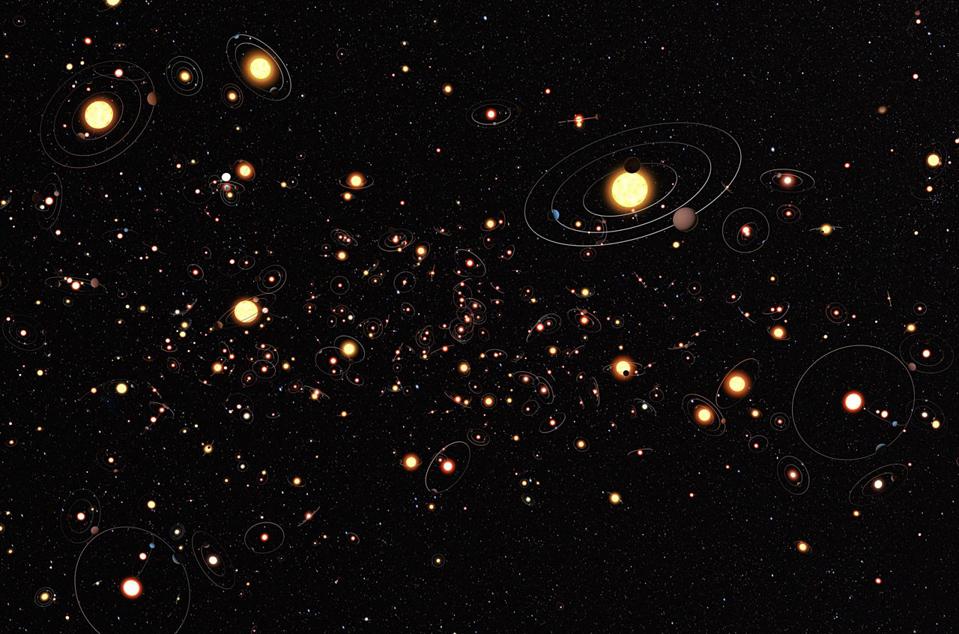
, , . , . , TESS , , , .
For example, Kepler's capabilities will not be enough to find an Earth-sized planet orbiting a star the size of the Sun or larger. Large stars have huge disks - it would take 12,000 Earth-sized planets to cover the Sun's disk, and Kepler is unable to detect a 1 / 12,000th decrease in brightness. In stars the size of the Sun, we can only find planets larger than our Earth - super-Earths. In the orbit of giant stars, we can only find gas giants.
If we want to find planets the size of the Earth or smaller - which are likely to be rocky and with little atmosphere - we will need to search for them in the orbits of the smallest stars - M-class stars, red dwarfs. The smallest planets usually revolve around such stars, but since they are very faint, it will be difficult for us to measure changes in their brightness. But:
- – . 80% – .
- , , .
- .
- 6% , , .

Comparison of the TRAPPIST-1 system and the inner planets of the solar system, as well as the moons of Jupiter. Although the classification of these planets may seem haphazard, there is an immutable connection between the formation and development of all these celestial bodies and their current physical properties. Planetary systems of red dwarfs are very similar to the magnified counterparts of Jupiter or Saturn with their moons.
It is important to understand that much of what we see through telescopes today is not equivalent to much of what exists in the universe. In any science, and especially in astronomy, we always lean towards those phenomena that are optimized to detect by our detectors, instruments and current capabilities. The easiest results to obtain do not necessarily reflect the real picture.
For a long time, the most common type of planet was "hot Jupiter". Now, it seems, worlds the size of Neptune are more common, and mini-Neptunes are even more common. We have not found worlds the size of Earth and smaller in sufficient numbers, but this has more to do with the limitations of the telescopes we have created than anything else. Extrapolating from our knowledge, we can say that the most typical type of planets will be rocky planets the size of the Earth or smaller, orbiting red dwarfs. It turns out that the Sun is not a typical star, and our planet is not a typical planet. Until we create tools suitable for finding them, such as the LUVOIR mission under development . - we will not be able to verify and confirm or refute our suspicions with the accuracy corresponding to scientific standards.