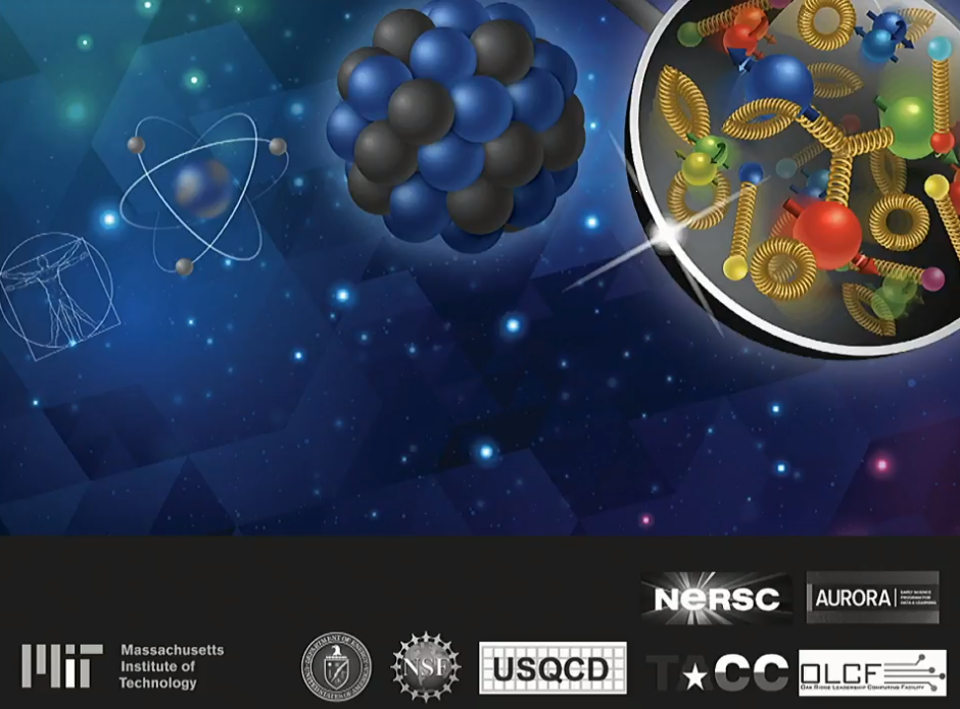
The smaller we study, the more fundamental knowledge about nature is revealed to us. If we could understand and describe the smallest existing objects, we could build on this basis an understanding of the large ones. However, we do not know if there is a limit to minimizing space.
There are rules in our Universe that we have never observed breaking. We expect some of them have never been violated. Nothing can travel faster than light. When two quanta interact, energy is always conserved. You cannot create or destroy momentum and angular momentum. Etc. But some of these rules, although we didn't see it, must have been broken at some point in the past.
One of them is the symmetry of matter and antimatter. Each interaction in which particles of matter are born or destroyed, destroys or generates an equal number of their counterparts from the world of antimatter - antiparticles. Considering that our universe is almost entirely made of matter, and contains almost no antimatter (there are no stars, galaxies, or stable cosmic structures made of antimatter), this symmetry has obviously been broken in the past. However, how exactly this happened remains a mystery. The mystery of the matter / antimatter asymmetry remains one of the greatest open questions in physics.
In addition, we usually say "particles", meaning the constituent parts of matter, and "antiparticles", implying the constituent parts of antimatter, but this is not entirely true. Particles are not always matter, and antiparticles are not always antimatter. This is what science has to say about this counterintuitive situation.
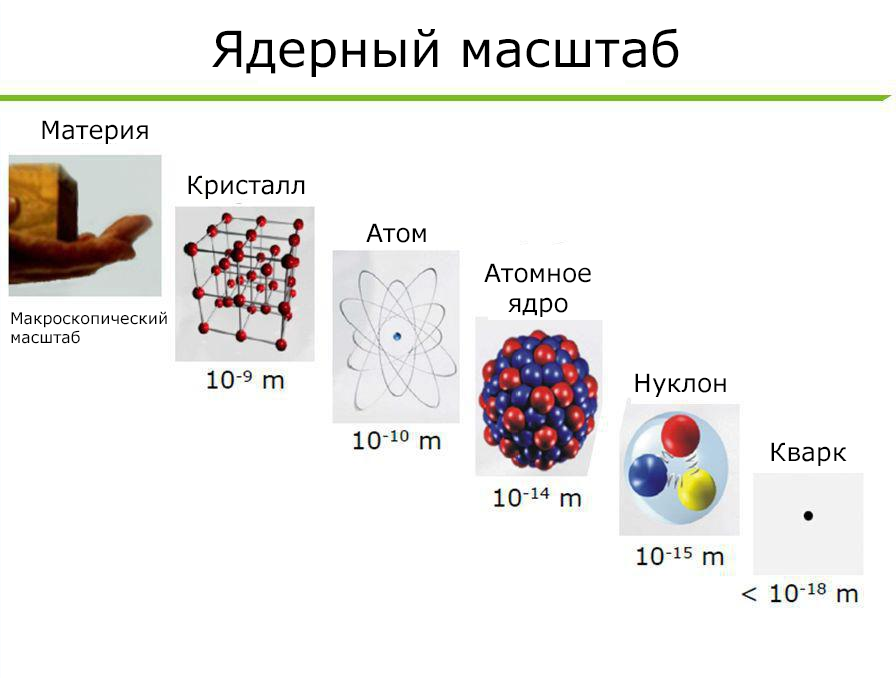
From macroscopic to subatomic scales, the size of fundamental particles plays a small role in determining the size of composite structures. It is not yet known if these building blocks are truly fundamental and point particles, but we understand how the universe works from large, cosmic scales to small, subatomic ones. The human body contains about 10 28 atoms.
Imagining the materials that can be found on Earth, you will probably assume that 100% of them consists of matter. This is roughly how it is - almost all of our planet is made of matter. It also consists of protons, neutrons and electrons - and all these are particles of matter. Protons and neutrons are compound particles made up of up and down quarks that bind together with gluons and form the nuclei of atoms. Electrons are attached to these nuclei - so that the total electric charge of the atom is zero, and the electrons are bound to the nuclei by electromagnetic interaction transmitted through the exchange of photons.
However, periodically one of the particles in the atomic nucleus undergoes radioactive decay. Typical example is beta decay... One of the neutrons turns into a proton, emitting an electron and an electron antineutrino. By studying the properties of the various particles and antiparticles involved in this decay, you can learn a lot about the universe.

Schematic representation of nuclear beta decay in a massive atomic nucleus. Beta decay works through weak interactions, converting a neutron into a proton, an electron, and an electron antineutrino. Before the discovery of neutrinos, it seemed that energy and momentum were not conserved in beta decays.
The neutron we started with has the following properties:
- It is electrically neutral, its total charge is zero.
- It consists of three quarks - two lower (with electric charges of -1/3) and one upper (with an electric charge of 2/3).
- It contains about 939 MeV energy in the form of a rest mass.
The particles into which it decays - a proton, an electron and an electron antineutrino - also have their own unique properties.
- The electric charge of a proton is +1, it consists of one down and two up quarks, and it contains about 938 MeV of energy in its rest mass.
- The electric charge of an electron is -1, it is a fundamentally invisible particle, and it stores about 0.5 MeV of energy in its rest mass.
- An electron antineutrino has no electric charge, it is a fundamentally invisible particle, its rest mass is unknown (but greater than zero), and no more than 0.0000001 MeV of energy is stored in it.
All binding conservation laws are still there. The energy is conserved, and a small supply of "extra" neutron energy is converted into kinetic energy of the resulting particles. The momentum is conserved, and the sum of the momenta of the resulting particles is always equal to the initial moment of the neutron. However, we want to not only study where we started and where we ended up - we want to know how it happened.

Free neutrons are unstable. They have a half-life of 10.3 minutes, and they decay into protons, electrons and electron antineutrinos. If you change a neutron to an antineutron, then all the particles will change to the corresponding antiparticles. Matter will be replaced by antimatter, and antimatter by matter.
According to quantum theory, decay requires a particle that controls it. In the quantum theory of weak interactions, which describes this process, this is done by the W - boson , which plays the role of one of the lower quarks of the neutron. Let's see what happens to fundamental particles.
One of the down quarks in a neutron emits a virtual W - boson, which turns into an up quark. In this interaction, the number of quarks is conserved.
Virtual W -a boson can decay into many different particles, but this process is limited by the law of conservation of energy. Its final decay products should not have more energy than the difference in rest mass between a neutron and a proton.
Therefore, for the most part, an electron is born in decay (to carry away a negative charge) and an electron antineutrino. In rare cases, you can see radiative decay, which results in an additional photon. In principle, it is possible to force the W - boson to decay into a combination of quarks and antiquarks (for example, from down and anti-up), but this requires too much energy - more than is obtained when a neutron decays to a proton.

Under normal conditions of low energies, a free neutron decays into a proton through weak interaction - in this case, the time increases upward in the diagram. At sufficiently high energies, this reaction can go in the opposite direction. A proton and a positron or neutrino can interact to produce a neutron - that is, when a proton interacts with a proton, a deuteron can appear. This is how the first critical synthesis step in the Sun works.
Now let's flip everything mirrored, going from matter to antimatter. Instead of the decay of a neutron, let's imagine the decay of an antineutron. The properties of the antineutron are very similar to the properties of the neutron mentioned earlier, but there are also important differences:
- It is electrically neutral, its total charge is zero.
- β ( +1/3) ( -2/3).
- 939 .
Moving from matter to antimatter, we simply replaced all particles with their antimatter counterparts. The masses remained the same, the composition (taking into account the "anti" prefix) remained the same, and the electric charge changed to the opposite. And although the neutron and antineutron are electrically neutral, the charge of their components has changed.
And this, by the way, can be measured! Although the charge is neutral, the electron has a so-called. magnetic moment , for which both spin and electric charge are needed. We were able to measure its magnetic moment - it is equal to -1.91 Bohr magnetons . The magnetic moment of the antineutron is +1.91 Bohr magnetons. All of its charged filling should be the opposite of matter and antimatter.

Thanks to experiments and new theoretical studies, we began to better understand the internal structure of nucleons, protons and neutrons, including how the "sea" of quarks and gluons is distributed. Studies allow us to explain most of the mass of baryons, as well as their nontrivial magnetic moments.
When decaying, an anti-lower quark emits a W + boson, a twin of W - an antimatter boson, which turns the anti-lower quark into an anti-upper one. Boson W +as before, virtual - it cannot be observed, and there is not enough mass / energy to create a "real" boson. However, its decay products are visible - a positron and an electron neutrino. (Yes, radiation effects can also appear - in rare cases, one or more photons are added to the decay products). Everything turns out to be a mirror image of the previous version, each particle of matter changes to a double of antimatter, and particles of antimatter (such as electron antineutrinos) - vice versa.
As for the materials that can be found on Earth, almost all of them are made of matter - protons, neutrons and electrons. A small part of these neutrons decays, which means that we also have W -bosons, extra protons and electrons (and photons), and some electron antineutrinos. Everything we know is well described by the Standard Model, and there are enough particles and antiparticles to describe everything. [clickable] The Standard Model helps us determine which particles exist in reality and which antiparticles for each of them. And although the Universe mainly consists of matter, and has only trace inclusions of antimatter, not every particle of it can be attributed only to either matter or antimatter.

We could replace Earth with "anti-Earth," an anti-material version of ourselves. Then we would simply replace each particle with its corresponding antiparticle. Instead of protons and neutrons (consisting of quarks and gluons), we would have antiprotons and antineutrons (consisting of antiquarks, but with the same 8 gluons). Instead of decay of neutrons via the W - boson, there would be decay of the antineutron via the W + boson. Instead of getting an electron and an electron antineutrino (and sometimes a photon), we would get a positron and an electron neutrino (and sometimes a photon).
Normal matter in the Universe consists of quarks and leptons. Quarks make up protons and neutrons (and baryons in general), and leptons include electrons and their heavier relatives, as well as three ordinary neutrinos. On the reverse side, there are antiparticles that make up antimatter - antiquarks and antileptons. Although ordinary decays follow different paths involving the W - and W + bosons , there is a small amount of antimatter in the form of positrons and electron antineutrinos. This would be so even if we could somehow "get rid" of the entire external Universe, including the Sun, cosmic rays and other sources of particles and energy.

Particles and antiparticles of the Standard Model, whose existence is predicted by the laws of physics. Quarks and leptons are fermions and matter. Antiquarks and antileptons are antifermions and antimatter. However, bosons are neither matter nor antimatter.
But what about the rest of the particles and antiparticles? When we talk about matter and antimatter, we are only talking about fermions - quarks and leptons. However, there are also bosons:
- 1 photon, an intermediary in electromagnetic radiation.
- 8 gluons, mediators in the strong nuclear force.
- 3 weak bosons, W + , W - and Z 0 , mediators in weak interactions and weak decays, as well as the Higgs boson, which is different from all the others.
Some of the particles are antiparticles to themselves - the photon, the Z 0, and the Higgs boson. W + is an antiparticle for W - , and three pairs of gluons are clearly antiparticles for each other (with the fourth pair, everything is a little more complicated).
If you collide a particle with its antiparticle, they annihilate, and can give out everything that is enough energy, taking into account all the quantum conservation laws - energy, momentum, angular momentum, electric charge, baryon number, lepton number, lepton family number, etc. etc. This is also true for particles that are antiparticles to themselves.

An equosymmetric collection of matter and antimatter bosons (X and Y, and anti-X with anti-Y) with the correct GUT properties could give rise to the asymmetry of matter and antimatter that we see in the Universe today.
It is noteworthy here how the idea of ββopposition of "matter" and "antimatter" appears. If you have a positive baryon or lepton number, you are matter. If negative, you are antimatter. And if you do not have a baryon or lepton number, you are neither matter nor antimatter! Although there are two types of particles - fermions (quarks and leptons) and bosons (everything else) - in our universe only fermions can be matter or antimatter.
If neutrinos turn out to be Majorana fermions, the theory will have to be revised - after all, Majorana fermions can be antiparticles for themselves.
This means that compound particles, such as pions or other mesons, consisting of combinations of quarks and antiquarks, do not belong to matter or antimatter - they consist of both. Positronium - an electron and a positron bound together, also does not refer to matter or antimatter. If there are leptoquarks or superheavy X or Y bosons from grand unification theories, then they will be an example of particles with both baryon and lepton numbers - for them there will be options from both matter and antimatter. If the theory of supersymmetry were correct, we would have fermionic counterparts of photons - photinos - not related to matter or antimatter. We could even have supersymmetric bosons - squarks - and then their versions of particles and antiparticles would be divided into matter and antimatter. Standard Model particles and their supersymmetric counterparts. A little less than half of them were found, and no one has yet seen evidence of the existence of the rest. Supersymmetry should improve the Standard Model, but has yet to make any successful predictions.

It would be very simple to consider that in the Universe there is matter, consisting of particles, and antimatter, consisting of their counterparts-antiparticles. This is partially true - most of the particles in the Universe are made up of what we think of as matter. If we replace them all with antimatter, we get what we think of as antimatter. This is the case for all quarks (with a baryon number +1/3), leptons (with a lepton number +1), antiquarks (with a baryon number -1/3), and antileptons (with a lepton number -1).
But everything else - all bosons that do not have baryon and lepton numbers, all composite particles, the total baryon and lepton numbers of which are equal to zero, are in the intermediate region, not belonging to matter or antimatter. In this case, one of their types cannot be attributed to a particle, and the other to an antiparticle. Yes, W +and W - can annihilate, like a particle / antiparticle, but they cannot be divided into matter and antimatter, like all other bosons. They, so to speak, cannot claim such a status. There is no point in asking which of them is matter and which is antimatter. For each other, they are particle and antiparticle, but none of them have the properties characteristic of matter or antimatter.