
So you can imagine a set of independent universes, not united by cause-and-effect relationships in the ever-expanding cosmic ocean. The idea of a multiverse comes from studying the theory of cosmic inflation in a quantum universe, but it's hard to prove.
Despite all our knowledge regarding the Big Bang, one of the greatest scientific mysteries remains the question of the appearance of the universe with exactly the properties that we observe in it. We understand how our modern universe has evolved from a hotter, denser and more homogeneous state. We understand how this state arose out of an earlier period of cosmic inflation. But if we go back in time far enough, at some point we will lose the ability to measure the properties that existed then or find any traces of early processes. We are left with only equations and assumptions. And one of the predictions, which appeared on the basis of a theoretical study of those very early times, is that our Universe is just one of many universes that make up a single the multiverse . But where will the mass and energy come from for the multiverse? This is what the reader is asking:
I don’t understand how to explain the mass of the multiverse. If it is constantly splitting into new universes, then how does the law of conservation of energy work? Is it because gravity is negative energy? Is it because expansion generates new energy? I'm sure I'm missing some elementary thing, but ... Where can I get enough mass for so many universes?
This is a very deep question, and the best answer to it will be full of surprises.
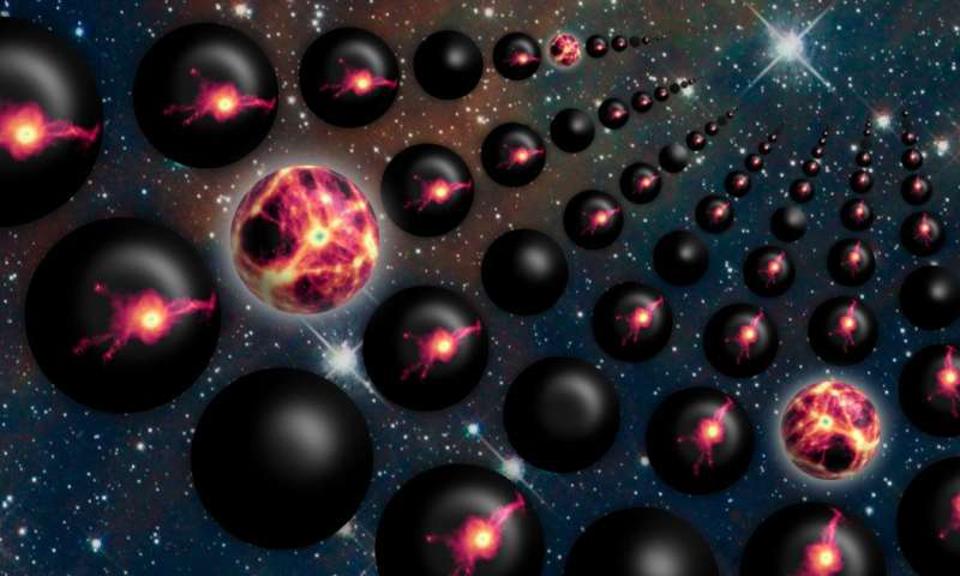
Universes of many possible types could appear in the multiverse. Some of them are suitable for a life like ours, and some may not. In the context of an expanding universe, the existence of the multiverse is inevitable, but understanding it in terms of energy is rather difficult.
Most people, when thinking about the multiverse, imagine a huge - possibly infinite - number of universes that appeared some time ago. Our Universe there is just one of many. Moreover, we ourselves are able to observe only a small part of our universe. The observable portion of the Universe stretches 46 billion light years from our location in all directions.
At the border of what we see, we do not notice anything unusual. But it exists due to the limited speed of light, and the amount of time that has passed since the Big Bang in our Universe. Therefore, we are not able to say exactly how far our Universe extends beyond what we see. It can continue even further over huge immeasurable distances, it can even be infinite in all directions. But it may turn out to be limited, just this border will lie beyond the limits of our cosmic horizon. No matter how much we wait, the amount of space available to our research will always be limited.

. , – . «» . 46 .
Fortunately, by studying what we can see, we can imagine what lies beyond the boundaries of what is available. Although the Universe is expanding, and all signals passing through it are limited by the speed of light, we have several interesting "milestones" available to us, hinting at what lies at a certain distance from us. We exist in the present, 13.8 billion years after the Big Bang. We live in a universe expanding at a measurable speed of the order of 70 km / s / Mpc. That is, each megaparsec (about 3.26 million light years) separating us from another object, on average, adds about 70 km / s to its speed relative to us.
We can say a lot about these cosmic limitations given everything we know about the energetic content of the universe. Namely - 68% dark energy, 27% dark matter, 4.9% ordinary matter, 0.1% neutrinos and 0.01% photons (that is, light).
- We will never be able to reach galaxies more than 18 billion light years away - even if we fly to them today at the speed of light.
- The light of the Big Bang will reach an object located 46 billion light years away from us today - just as we see this light emanating from the point as it was 13.8 billion years ago.
- An object located at a distance of 61 billion light years from us today is not visible to us, but when the light from it reaches us, it will be the farthest object we observe.

() () . , 13,8 , , , 46 – , . , 61 . , 135% .
These are the boundaries of only the universe we observe. We do not know how far the rest of the unobservable part of it extends after the same Big Bang. But, of course, we can impose restrictions on it. If the Universe is somehow closed on itself - in the form of a loop, or repeats itself in some other way - then the scale of this repetition is greater than the part that we see today. If it is not closed, the restriction on the curvature of space (and it should be less than ~ 0.002% of the energy density of the Universe) suggests that the Universe should extend at a distance at least 400 times larger than the part we see in all directions. That is, its volume should be at least 64 million times larger than the volume of the Universe we observe. And in principle, the universe can be infinite in general.
But, no matter how huge our Universe is, this does not mean that it is so alone. Even if it is infinite, there may be others - remember that infinities have different powers .
The main thing in this case is to understand where the physical idea of the multiverse came from. It appears when you take the idea of cosmic inflation seriously. And this, in turn, is the best theory and mechanism to date, explaining what happened before the Big Bang, how everything led to it and gave birth to it.

Quantum fluctuations that appear during inflation are stretched over the entire Universe, and at the end of inflation they become fluctuations in the density of matter. Over time, this leads to the appearance of large-scale structures, as well as temperature fluctuations observed in . .
Trying to extrapolate back in time the processes that took place at the beginning of the Big Bang, based on today's observations, we come across several mysterious phenomena. We see that in any direction in the Universe, on average, the same matter density and temperature are preserved. At the same time, the opposite parts of the Universe, remote from each other, did not have time to exchange information for the entire known history. We see that the total energy density and the original expansion rate should have been the same at the beginning of the hot Big Bang to within 25 significant digits after the decimal point - and this the Big Bang does not explain. We do not see high-energy traces of the early Universe, the existence of which could be expected if infinitely high temperatures and densities were observed in the early stages of its development.
How is this possible? Hence the idea of cosmic inflation arises: perhaps there was a phase in the history of the Universe that preceded the Big Bang. In this phase, the Universe was not filled with particles, antiparticles, radiation and other quantizing forms of energy as it is today. It was filled with some kind of energy, reminiscent of dark energy - an energy inherent in space-time itself. In this state, the universe is relentlessly expanding at an exponential rate. And only when this expansion stops, energy turns into particles, antiparticles, and radiation - the Big Bang occurs.
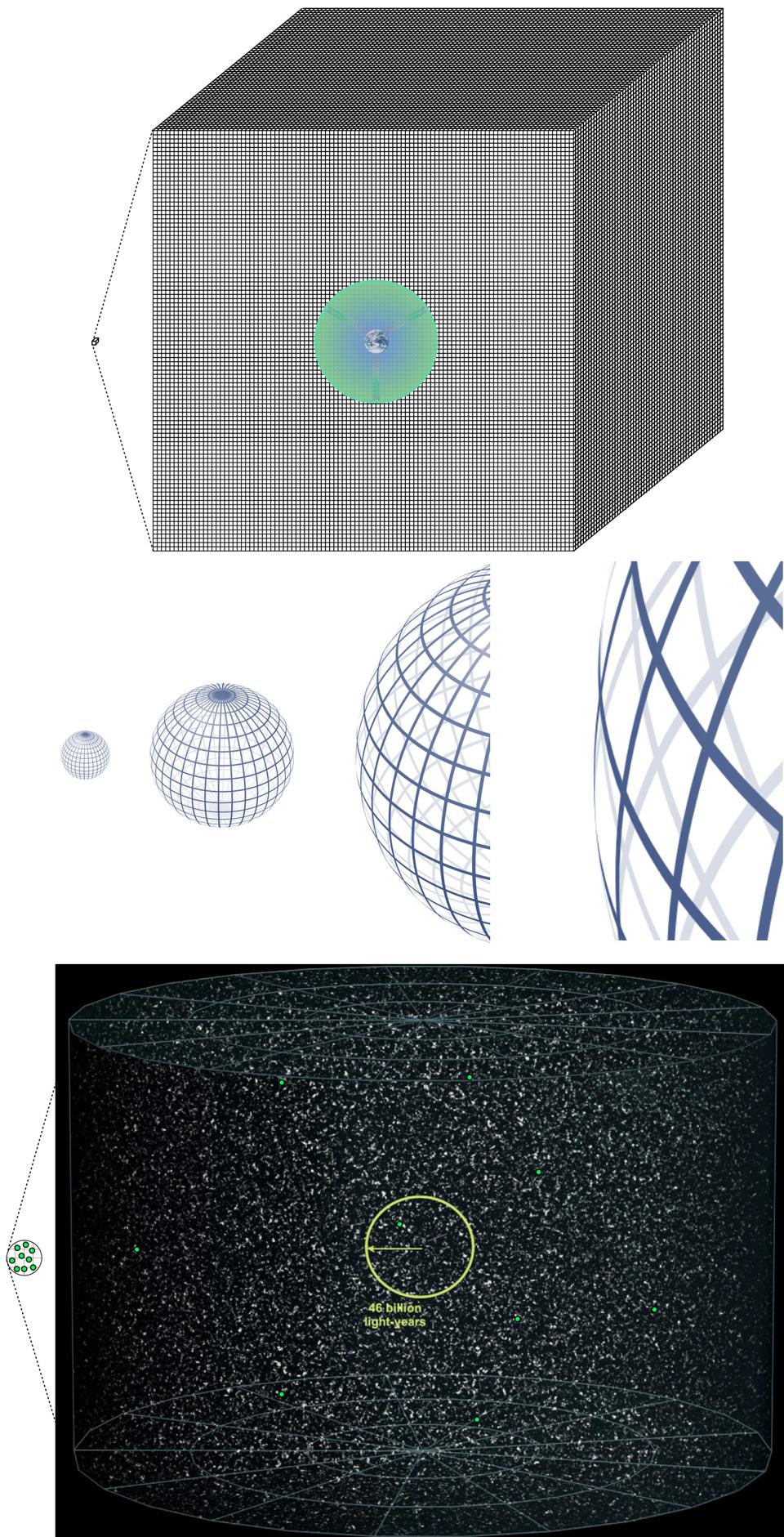
, . , , - . , (), ( ), - ().
This is one of the biggest ideas of today's cosmology, and one of the most successful both in explaining the phenomena we observe and in predicting new ones that we were later able to test. The Universe has the same properties in all directions because it emerged from a piece of space that once belonged to a single region, stretched to enormous sizes by inflation. There is a balance between energy density and spatial curvature because these properties were determined by the dynamics of inflation, forcing them to be in balance. And there were no high-energy relics left because the universe never reached arbitrarily high temperatures - they were limited by the energy scale of inflation.
If inflation was a quantum field, it must be subject to quantum fluctuations. And it was inevitably such a field, given that in the universe (probably) everything is fundamentally quantum in nature. Energy fluctuations create regions of increased density from which galaxies are made, as well as regions of lower density, which turn into cosmic voids . Inflation can be thought of as a ball rolling from the top of a very flat hill to the bottom. From quantum fluctuations follows the existence of "pockets" of the inflationary universe, in which inflation ends earlier than in other places. And there must also be places where inflation has not ended today.

Above: Inflation ends when the ball rolls to the bottom.
: , .
: (, , ) , (, ) – , .
When inflation ends, there is a hot Big Bang and a new chance for a universe like ours. It doesn't matter where or when it happens, and it doesn't matter if inflation continues in the surrounding regions. We don't know much about the many of these universes, even in theory. But if the inflationary theory is correct, and the laws of physics continue to work during inflation, then the existence of these universes is inevitable. This is where the idea of a multiverse comes from - from a purely physical point of view, without references to philosophy, interpretations of quantum mechanics, or to the universe as it was before inflation.
This is where the idea of the universe emerged from nothing. If by "nothing" we mean empty space that appeared during inflation, then it will give rise not only to a universe like ours, but also a huge (and possibly infinite) number of other independent universes. Each of them will be filled with its own particles, antiparticles, radiation and other allowed forms of energy.
But, despite all this wonderful story, you may still be concerned about the question - where does the energy for all this come from?
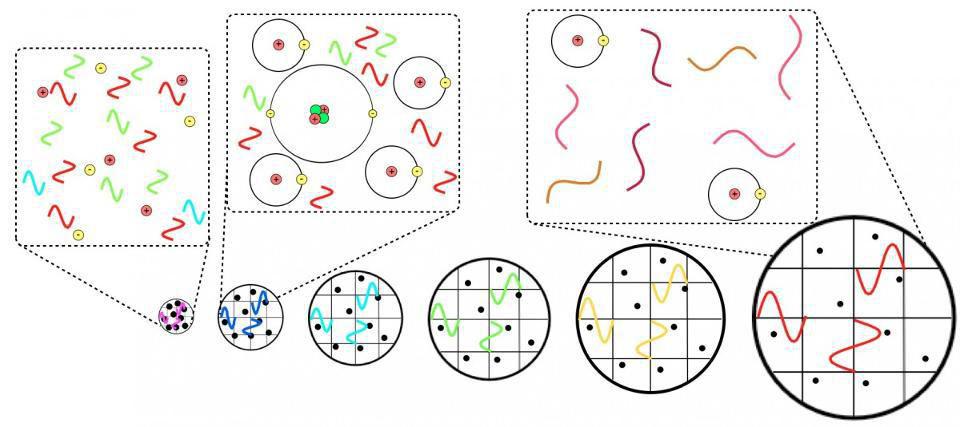
, , . , , . , , , . .
This is the point at which the processes begin to contradict our intuition. You, of course, have heard about the law of conservation of energy - that energy cannot be created or destroyed, and that it can only pass from one form to another. This is the case for any event in the Universe - be it before interaction, transformation, or any physical phenomenon that occurs in a certain place at a certain moment in time. Such an event can be a collision of two particles, a hit of light on a surface, a meeting of two observers in one place. As far as we know, in all events that have ever happened in the Universe, energy has been conserved.
But in the entire Universe as a whole, and in all space-time, energy is not always conserved or even precisely determined. Energy can be clearly defined in static space-time - not changing from one moment in time to another. An example of such a space is the vicinity of a black hole. Its properties do not change until the black hole changes its mass. However, an expanding or contracting universe changes over time. With the growth of space, the energy of different components changes in different ways, giving in to quantitative determination.
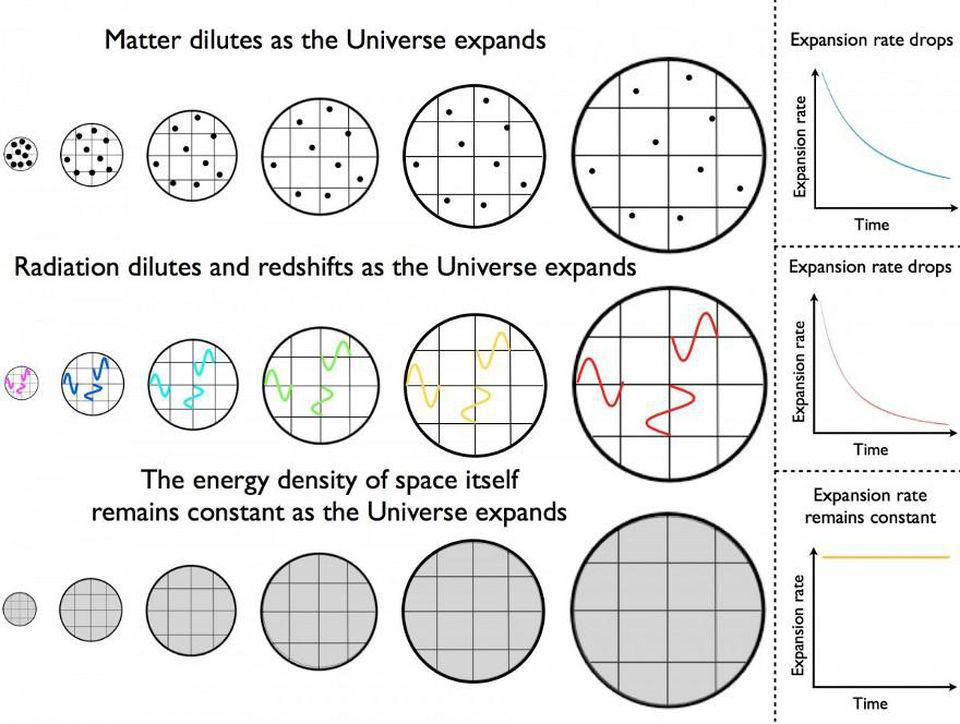
If the density of matter and energy in an expanding universe decreases due to an increase in its volume, dark energy is a form of energy inherent in space itself. New space is created in the expanding universe, while the density of dark energy remains constant.
Both normal and dark matter are made up of particles - they have a certain mass, they occupy a certain volume. With the expansion of the Universe, the number of particles does not change, but the volume increases, but the total energy remains constant.
Radiation behaves differently. The energy of light waves is determined by their length. The shorter the length, the higher the energy, and vice versa. With the expansion of the Universe, the number of radiation quanta does not change, but the wavelengths are stretched, due to which each quantum loses its energy. With the passage of time and the increase in volume, the total energy decreases.
Dark energy also behaves in its own way. This is the energy inherent in the very fabric of space. Its value today is extremely small, but during inflation it was huge. With the expansion of space, the energy density does not change, but the volume increases. The total energy of the universe increases over time, as it is considered as energy density times volume.

We are used to having positive pressure inside different objects. Dark energy in this case is counterintuitive, since its pressure is negative, but at the same time it makes the fabric of space expand.
Many people do not like this, but in fact, in the Universe, the space of which expands or contracts with time, energy is not conserved, and is not even determined accurately. You can make it persist by postulating a global definition of energy, in which you isolate a part of the universe and require that energy be conserved within its boundaries. This can be done only by introducing another definition - the work done over the border you drew as the Universe expands. Radiation does positive work by losing energy. Dark energy (energy of inflation) does negative work, increasing the overall energy.
For all its attractiveness, this approach cannot be called reliable. We can choose it by a volitional decision, only in order to satisfy our ideas about the need to save energy. But in fact, the conservation law works only in a certain place in space, and not for the entire expanding Universe. You may have heard this expression: there is no free lunch. It may not happen on Earth, but this does not apply to the expanding Universe. If the ideas about inflation and the multiverse are correct, then perhaps the entire universe is a giant free lunch. In our difficult times, one can be grateful at least for this fact.