The Parisian team of researchers carried out the most accurate measurement of the fine structure constant to date, taking away our hope for the existence of an unknown interaction in nature.

The fine structure constant was introduced in 1916 to quantify the spacing between two lines in the spectrum of colors emitted by certain atoms. In the photo, densely located frequencies are visible through the Fabry - Perot resonator.
Among the fundamental constants, the most famous is the speed of light c. However, its numerical value does not tell us anything about nature - it depends on the units of measurement, be it meters per second or miles per hour. On the other hand, the fine structure constant has no dimension. This is a sheer number that has a tremendous impact on the universe. Richard Feynman wrote that this is "a magic number that we found without any understanding." Paul Dirac considered the origin of this number "the most fundamental of the unsolved problems in physics."
The value of the fine structure constant, denoted by the Greek letter α, is very close to the fraction 1/137. It is often found in equations describing the behavior of light and matter. “In architecture, for example, the golden ratio is common,” said Eric Cornell , a physicist at the University of Colorado and the National Institute of Standards and Technology and a Nobel laureate. “And in the physics of low-energy matter - atoms, molecules, chemistry, biology - there is a constant ratio of sizes of larger to smaller. And these ratios often turn out to be degrees of constant fine structure. "
The constant is ubiquitous because it describes the strength of the electromagnetic force acting on charged particles - electrons and protons. “In the everyday world, we are faced with either gravity or electromagnetism. This is why alpha is so important, ”said Holger Mueller , a physicist at the University of California, Berkeley. 1/137 is not much, and the influence of electromagnetism is weak. As a result, charged particles form predominantly empty atoms, in which electrons move in distant orbits and are easily torn off from them. This leads to the formation of chemical bonds. On the other hand, the constant is large enough - if it were closer to 1/138, carbon would not be created in the stars, and the life familiar to us could not appear.
For almost a century, physicists have been obsessed with the desire to find out why the value of alpha turned out to be this way. But today they have practically lost interest in this issue, and admit that the value of the fundamental constants can be random, determined by the roll of the cosmic dice at the birth of the universe. Now they have a new goal.
Physicists want to measure the value of the fine structure constant as accurately as possible. Since it is found everywhere, its precise meaning allows them to test their theory of the relationship of elementary particles. Namely, a magnificent set of equations known as the Standard Model of Particle Physics... Any discrepancy in precisely measured values related to each other could indicate the existence of new particles or effects not accounted for by the standard equations. Cornell calls such precise measurements the third way to experimentally uncover the fundamental principles of the universe - after particle accelerators and telescopes.
In December 2020, a team of four physicists led by Saida Gelati-Khalifa of the Kastler-Brossel laboratory in Paris published in Nature a new paper with the most accurate measurement of the fine structure constant to date. The team received the value of a constant up to 11 digits: α = 1 / 137.035999206.
With an error of only 81 trillion, the new value is three times more accurate than the previous one , carried out in 2018 by the team's main competitor, the Mueller group in Berkeley. Before Müller, Gelati-Khalifa received the most accurate value at that time in 2011. Of the new value received by their rivals, Müller said: “Three times is serious. You can call it an excellent achievement without much hesitation. "
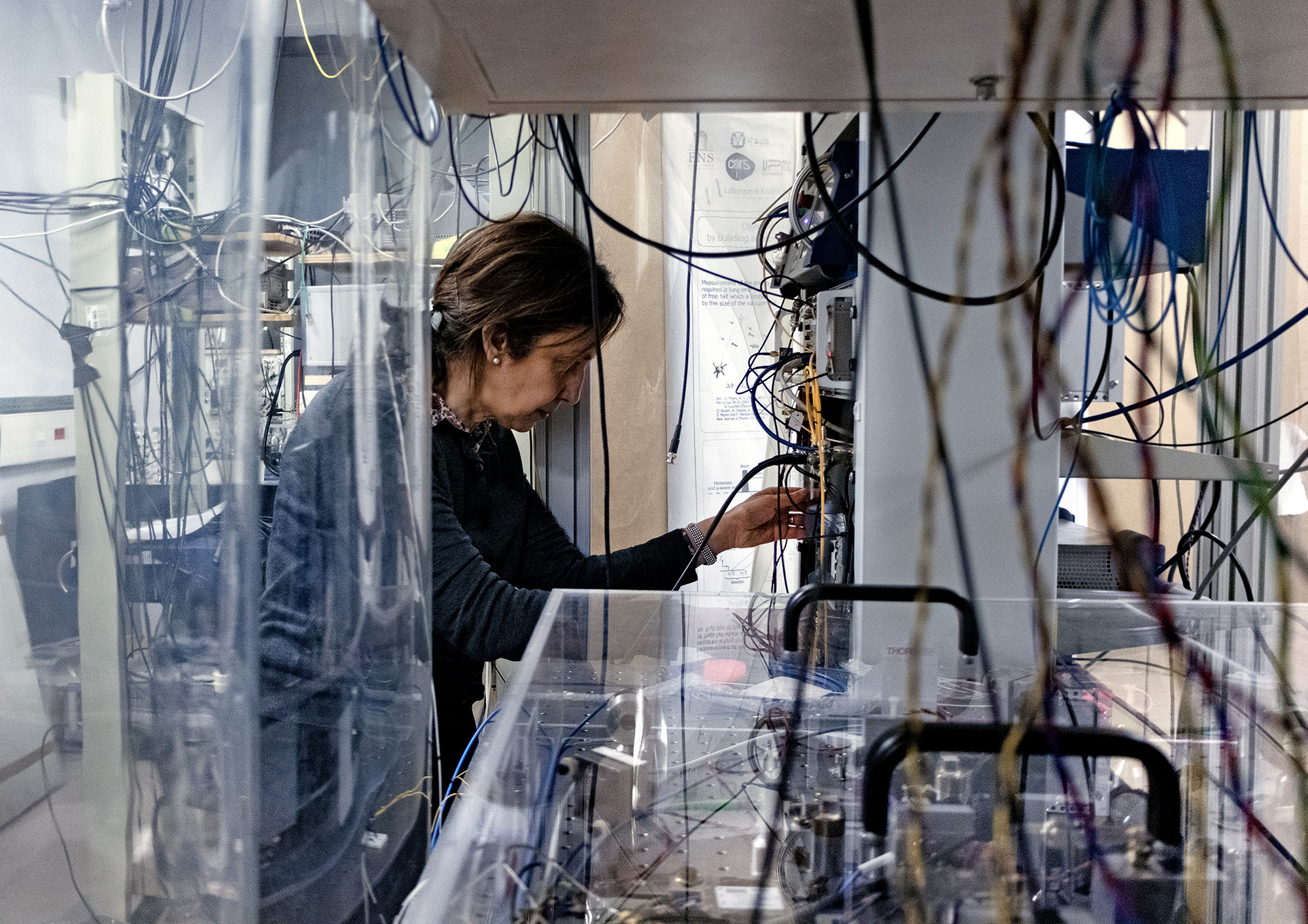
Saida Gelati-Khalifa in her Paris laboratory
Gelati Khalifa has been improving her experiment for 22 years. It measures the fine structure constant by measuring the amount of deflection of rubidium atoms when they absorb a photon. Müller does the same with cesium atoms. The deflection rate characterizes the mass of rubidium atoms - in a simple formula for the fine structure constant, this is the most difficult term to measure. “The bottleneck is always the least accurately measured value, so any improvement in this area leads to a refinement of the fine structure constant,” explained Müller.
The Parisian experimenters began by cooling the rubidium atoms to almost absolute zero and then throwing them into a vacuum chamber. While the cloud of atoms was descending, the researchers, using laser pulses, brought them into a quantum superposition of two states - colliding with a photon, and not colliding. The two likely versions of each atom moved along separate paths until new laser pulses brought the halves of the superposition back together. The more the atom deflected when colliding with a photon, the more it differed in phase from its other version, which did not collide with the photon. The researchers measured this difference by calculating the rate of deflection. “From the deflection rate we get the mass of the atom, and the mass of the atom directly determines the fine structure constant,” Gelati-Khalifa said.
In such precise experiments, every little thing counts. The first table of the new work shows the "error budget" - 16 sources of possible errors and inaccuracies that affect the final result. This includes gravity and the Coriolis force due to the Earth's rotation, both of which have been carefully calculated and taken into account in measurements. The biggest contribution to the error budget comes from the disadvantages of the laser, which scientists have been constantly improving for many years.
The hardest thing for Gelati Khalifa is to figure out when to stop and publish the results. He and the team stopped on February 17, 2020 - when the coronavirus was gaining momentum in France. When asked whether a scientist’s decision about when to publish a work is like an artist’s thoughts about when to consider a painting complete. Gelati Khalifa replied: "Exactly, precisely, precisely."
It was a surprise that the result of its measurements differs from the result of Mueller from 2018 in the tenth position after the decimal point - this difference is greater than the error of both measurements. This means that, with the exception of fundamental differences between rubidium and cesium, one or both measurements may contain an unaccounted for error. The Parisian group's measurement is more accurate, so it has an edge for now, but both teams will improve their experimental setup and try again.
Although the two measurements are different, they are close to the alpha value obtained from accurate g-factor measurements. electron - a constant associated with its magnetic moment, in fact, the torque that it experiences in a magnetic field. “The fine structure constant can be related to the g-factor through a huge set of formulas,” Cornell said. "And if there weren't enough physical effects in the equations of the Standard Model, we'd get the wrong answers."
But in fact, all measurements are in perfect agreement, which for the most part rejects some assumptions about the existence of new particles. The coincidence of the best measurement of g-factor and the measurement made by Mueller in 2018 was hailed as the greatest triumph of the Standard Model. Gelati Khalifa's new result is even better in line with expectations. “This is the best fit between theory and experiment,” she said.
And yet she and Mueller are determined to further improve the experiments. The Berkeley team switched to a new laser with a wider beam (which will make it possible to fire more evenly at the cloud of cesium atoms). The Parisian team plans to replace the vacuum chamber and make other improvements.
What kind of person does one have to be to spend so much energy on such a modest result? Gelati Khalifa named three properties: "You need to be scrupulous, ardent and honest with yourself." Mueller said, “I think this is all very interesting. Personally, I love building shiny new gear. And apply them to some important things. " He noted that no one can single-handedly build a high-energy collider like the European Large Hadron Collider. However, when assembling an ultra-precise instrument, "it is possible to carry out measurements that are important for fundamental physics, working only three or four of us."