
Try reading English-language sources on the history of chemistry and look for references to the periodic table. You will be surprised, but still make sure that this wording is carefully avoided. Persistently and somehow politically correct they write about the "periodic table of elements." With the mention not only of the periodic, but all those involved, emphasizing the role of Meyer , Dobereiner and Chancourtois with no less fervor than the determining role of opening a second front in the final stages of World War II.
Paying tribute to the respected Western partners of Mendeleev and personally to Robert Bunsen, with whom Dmitry Ivanovich studiedin 1859-1861, we note that Mendeleev went down in the history of science not as a classifier of the known, like Linnaeus, but as a visionary who was able to predict not yet discovered elements and, more importantly in the context of this article, to correctly arrange iodine and tellurium, despite the fact that tellurium is heavier than iodine.
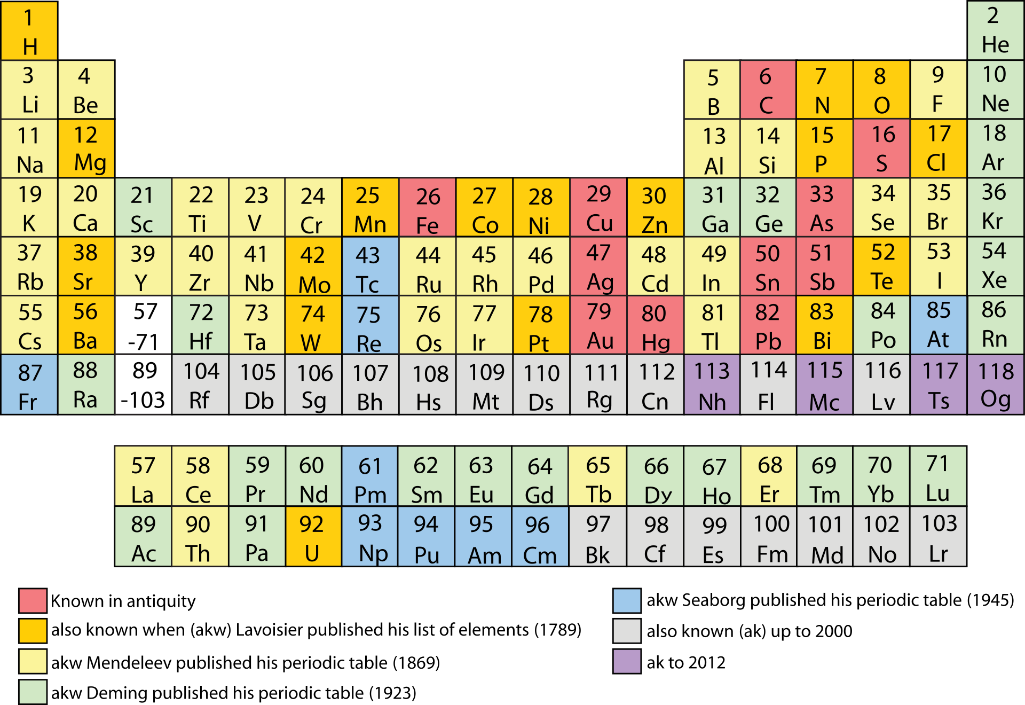
At present, Oganesson (Og) No. 118 closes the periodic table. It is located exactly under the radon (No. 86) and, according to Mendeleev's logic, should be a noble gas, since it closes the seventh period. But with the end of this most amazing, ephemeral and explosive period, which contains uranium, plutonium, mendelevium, flerovium and oganesson, the questions are again actualized: where does the periodic table end? And is the periodic law observed to its very limit? Surprisingly, the first answer to this question was given quite confidently by Richard Feynman.
In doing so, he relied on the traditional model of the atom proposed by Bohr. As you know, in Bohr's model, the atomic nucleus is surrounded by a cloud of electrons, and the electrons revolve around the nucleus only in strictly defined allowed orbits. An electron cannot occupy an intermediate orbit, but it can move from one permitted orbit to another. This transition occurs instantly with the emission or absorption of a quantum of energy and is called a "quantum leap".
The speed of an electron in a particular quantum state is calculated by the following formula

where
Z
is the atomic number corresponding to the number of protons in the nucleus of an atom and, accordingly, to the number of electrons orbiting a neutral atom. Here
n
Is the quantum state of the electron, and


where e is the elementary charge, h is Planck's constant, and e0 is the dielectric constant, also called the free vacuum permeability.
Accordingly, the further from the nucleus is the outer electron shell of the atom, the higher the speed of the electron moving along it. Richard Feynman calculated that at Z = 137 the speed of the electron will be slightly lower than the speed of light. If you follow this logic, the element with atomic number 138 cannot exist; otherwise, its outermost electron would exceed the speed of light.
Rutherfordium and lawlessness
However, in practice, things are more complicated. First, relativistic effects begin to appear in the nuclei of heavy and superheavy elements. Calculations predicting where the periodic table might end are based on the theory of relativity. With an increase in the nucleus, there are more and more protons in it, which means that the attractive force acting on the electrons also increases. Accordingly, the speed of the outermost electrons grows, increasingly approaching the speed of light. At such speeds, the electrons become "relativistic", and the properties of these elements cannot be fully explained by the position of the element in the table alone. Some of these effects are visible to the naked eye. So, in gold atoms, electrons revolve around the nucleus at about half the speed of light. Because of this, the outlines of the orbitals change sothat gold absorbs the blue part of the visible spectrum, and the rest of the photons are reflected from it. We observe white light minus the blue-violet component, and as a result, gold acquires a characteristic yellow-red sheen, which stands out against the background of the surrounding silvery metals.
Back in the 1990s, the first experiments were performed, showing that rutherfordium (104) and dubnium (105) exhibit properties other than those assigned to them in accordance with the positions in the periodic table. According to the periodic law, they should resemble in properties those elements that are located directly above them, respectively, hafnium and tantalum. In fact, rutherfordium reacts like plutonium, located quite far from it, and dubnium - like protactinium. On the other hand, seborgium (106) and borium (107) follow the law derived by Mendeleev.
Further more. It turns out that X-ray (111) approaches in properties with astatine, and not with gold, and copernicium (112) gravitates in properties to noble gases, even more than oganesson (118). Probably, tennessine (117) is more similar in properties to gallium, and nichonium (113) is comparable to alkali metals. All these anomalies are associated with an increasingly pronounced manifestation of relativistic effects in superlarge atoms.
A little about particle-wave dualism
Bohr's model of the atom in the interpretation, according to which the table should be closed by element No. 137, also does not fully correspond to the real state of affairs. The subject of quantum physics is much more complex than the subject of classical physics; as a rule, quantum phenomena have no visual analogue at the macro level. For example, in accordance with the laws of classical physics, electrons revolving around the nucleus must fall on the nucleus, and the atoms must collapse.
It would seem that the very existence of the atom is a refutation of the laws of physics. But in reality, everything is different. The classical laws are unshakable, but electrons do not fall on the nucleus, since, strictly speaking, an electron is not a particle. The electron obeys wave-particle duality, that is, it simultaneously exhibits the features of a particle and a wave, and therefore does not fall on the nucleus. Nevertheless, even taking into account the wave-particle duality, the speed of an electron cannot exceed the speed of light in a vacuum.
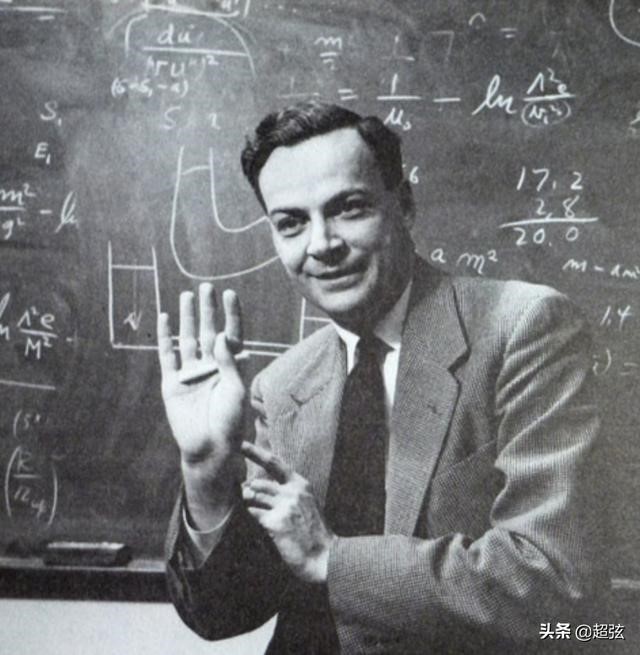
Mr. Feynman himself,
Richard Feynman believed that with an atomic number of more than
Z
= 137, a neutral atom cannot exist. The point is that, according to the relativistic Dirac equation, for large values
Z
the ground energy state of the electron closest to the nucleus will be expressed as an imaginary number. However, this reasoning assumes that the kernel is pointwise. If we assume that the core has a physical size, even if the minimum, but not zero, then the periodic table should continue until
Z
≈173.
What's next
It is believed that for
Z
≈ 173 1s-subshell under the influence of the electric field of the nucleus "plunges" into the negative continuum ( Dirac sea ), which leads to the spontaneous creation of electron-positron pairs and, as a consequence, to the absence of neutral atoms above the element Ust (Unseptrium) with
Z
= 173. Atoms with
Z > Zcr
173 are called supercritical atoms. It is also assumed that elements with
Z > Zcr
can exist only as ions.
Supercritical atoms cannot be fully ionized, since spontaneous pair production will violently occur on their first electron shell, in which an electron and a positron emerge from the Dirac sea, moreover, the electron is woven into the atom, and the positron flies away. True, the field of strong interaction surrounding the atomic nucleus is very short-range, so that the Pauli exclusion principle does not allow further spontaneous pair production after the filling of those shells that are immersed in the Dirac Sea. Elements 173-184 are called weakly supercritical atoms , since they have only a shell immersed in the Dirac Sea
1s
; it is assumed that the shell
2p1/2
will completely fill around item 185, and the shell
2s
- about element 245. So far, it has not been possible to experimentally achieve spontaneous pair production, trying to collect supercritical charges by colliding heavy nuclei (for example, lead with uranium, which could give
Z
= 174; uranium with uranium, which gives
Z
= 184, and uranium with californium, which gives
Z
= 190). Perhaps nuclear instability will play a key role in the final of the periodic table, rather than the instability of electron shells.
Finally, it is assumed that an
Z
entire continent of stability , consisting of hypothetical quark matter, may be hidden in the region > 300 (it is also quantum chromodynamic matter). Such matter can be composed of free up and down quarks, rather than quarks bound into protons and neutrons. It is assumed that this is the ground state of baryonic matter , which has a higher binding energy per baryon than nuclear matter. If such a state of matter is real, then perhaps it can be synthesized in the course of thermonuclear reactions of ordinary superheavy nuclei. The products of such reactions, due to the high binding energy, must completely overcome the Coulomb barrier.
So far, all this is theory, and we, we repeat, managed to fill only the 7th period of the periodic table by the 150th anniversary of the discovery of the Periodic Law (1869-2019). Either way, the half-life of new heavy elements is rapidly decreasing; if for Rutherfordium-267 it is about 1.3 hours, then for X-ray-282 it is only 2.1 minutes, and for Oganesson it is calculated in hundreds of microseconds. Thus, the ending is close, and after it a sequel or director's version of the material world may open. The way there lies through the Feynmania sublight orbitals.