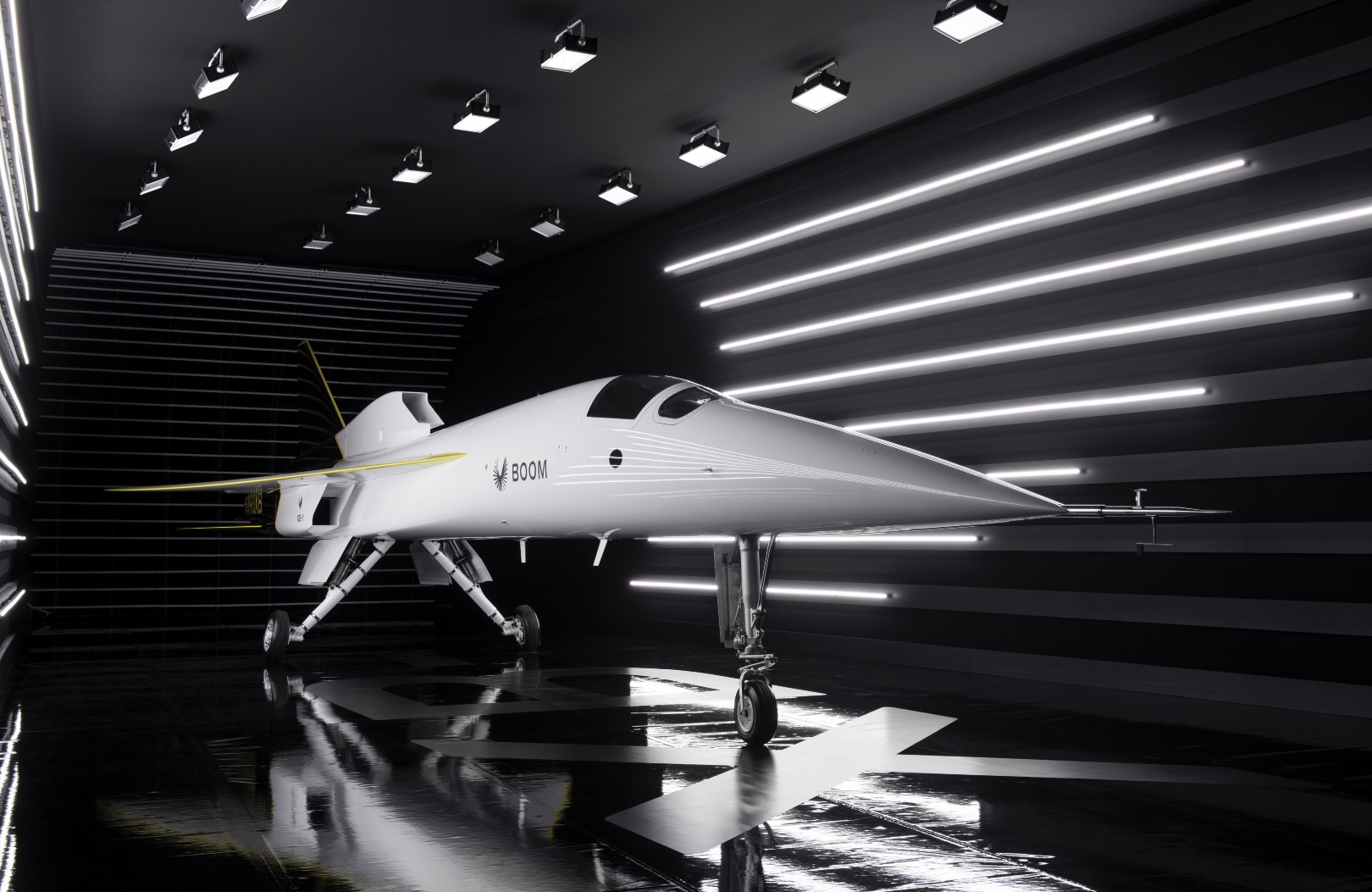
The XB-1 supersonic demonstrator, first left the hangar on October 7, 2020.
The founder and CEO of Boom Supersonic, Blake Scholl, is set to create a "SpaceX analog for the aviation industry" and, over time, cut the average intercontinental travel time in half. If the entrepreneur succeeds, the flight time from Tokyo to Seattle will be only 4.5 hours, and the cost will be comparable to modern business class.
The first ever independently developed supersonic jet designed for high speeds. From the nose of the aircraft to the delta wing and carbon composite frame, everything has been optimized and designed for supersonic cruising.
Following the presentation of the XB-1 on October 7, 2020, Boom Founder and CEO Blake Scholl conducted an orientation tour of the aircraft to highlight the key components of the XB-1's design.
Fuselage
One of the XB-1's most pronounced supersonic features is its hull. “The XB-1's 22-meter fuselage is designed for speed that reduces drag when flying at supersonic speeds,” Scholl said.
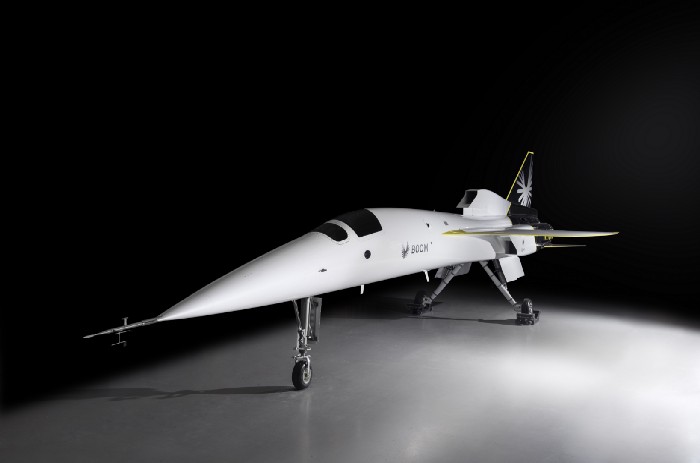
The long, thin tube fuselage features a high coefficient of fineness (the ratio between aircraft length and width) for maximum efficiency. Boom engineers designed the fuselage using both physical and digital tools such as scaled wind models and computational analysis to ensure the ideal shape was quickly recreated.
Housing
At supersonic speed on the outer surface of the aircraft, the temperature can reach 125 ° C. The XB-1 carbon composite frame withstands this heat and maintains its shape in the most extreme flight conditions.
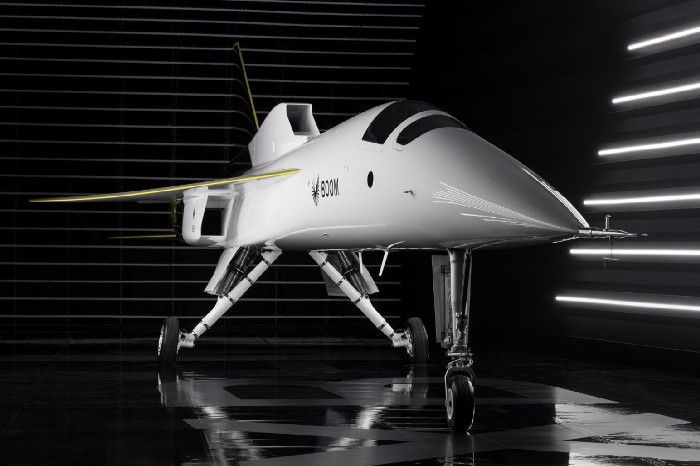
“The carbon composite frame retains its rigidity and strength even at high temperatures and high-speed flight pressures,” Scholl explained. By building the aircraft primarily from carbon fiber, the Boom has gained a number of significant advantages over traditional metals such as aluminum, which can expand by more than 25cm during supersonic flight.
Deltoid wing design
The XB-1's triangular wing design differs markedly from the subsonic aircraft we know today. Scholl explained: "The XB-1 delta wing balances the low speed performance during takeoff and landing at high speed."
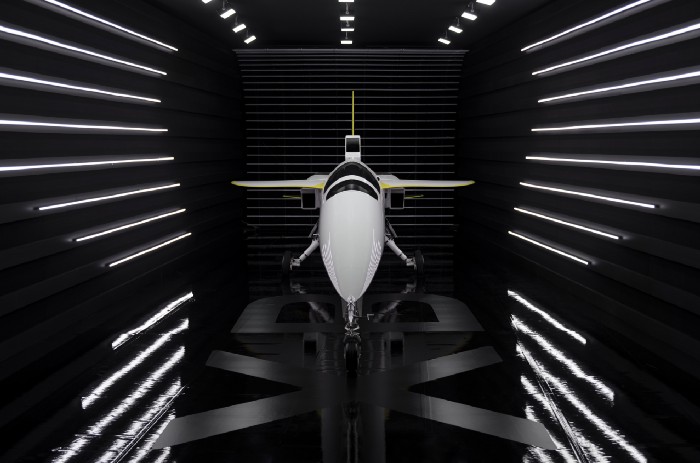
As strong as it is efficient, the unique wing design of the XB-1 has been safety tested at a maximum load of 27,000 kgf.
Power point
In the aft fuselage of the XB-1 there are three J85-15 engines providing a maximum thrust of 5600 kgf. “These three J85 engines, developed by General Electric, enable supersonic speeds,” added Scholl.

Cockpit
The single-seat XB-1 cockpit, located at the pilot's line of sight, provides visibility during takeoff and landing. The Forward Vision System installed in the A-pillar adds a second view of the runway during landing.
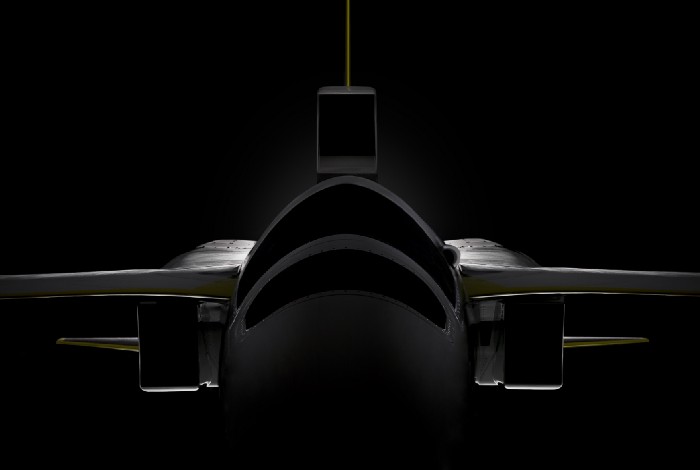
The involvement of Boom test pilots early in the design phase ensured that the cockpit and instrument configurations were tailored to their needs. The ergonomic cockpit was developed with input from our test pilots, Scholl said, with hundreds of hours of human factors analysis and usability testing.
Nose part
The nose, where high velocity air first meets the aircraft, is precisely shaped to control how the rest of the vehicle interacts with the airflow. It minimizes drag, improves low-speed performance and keeps all the components behind it working successfully.
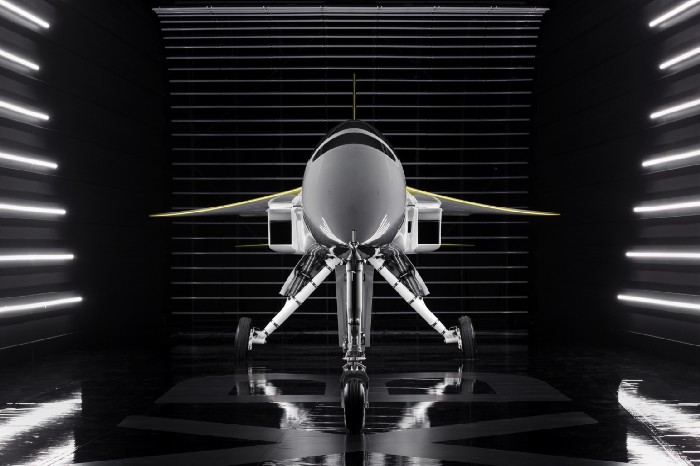
“The nose creates a precise vortex flow for stability over a wide range of flight speeds,” Scholl concluded.
Looking to the future
Each of the XB-1 components have been designed for safe and efficient supersonic flight. More importantly, all the experience gained during the creation of this aircraft directly influenced the design and development of the Boom Overture supersonic passenger airliner. The XB-1 continues to pave the way for a new era of massive supersonic travel.
Once the demonstrator has successfully passed a series of tests and trials, the engineering team will begin assembling the full-size Overture with all the blueprints ready. Overture is a next-generation aircraft designed for 100 passengers with business class comfort level. Scholl said Overture will cost $ 6 billion to develop - about 5.3 times cheaper than the Boeing 787 Dreamliner.
From canoes to assembled aircraft: the XB-1 approaches the taxiway
(original From 'canoe' to assembled aircraft: XB-1 approaches the taxiway )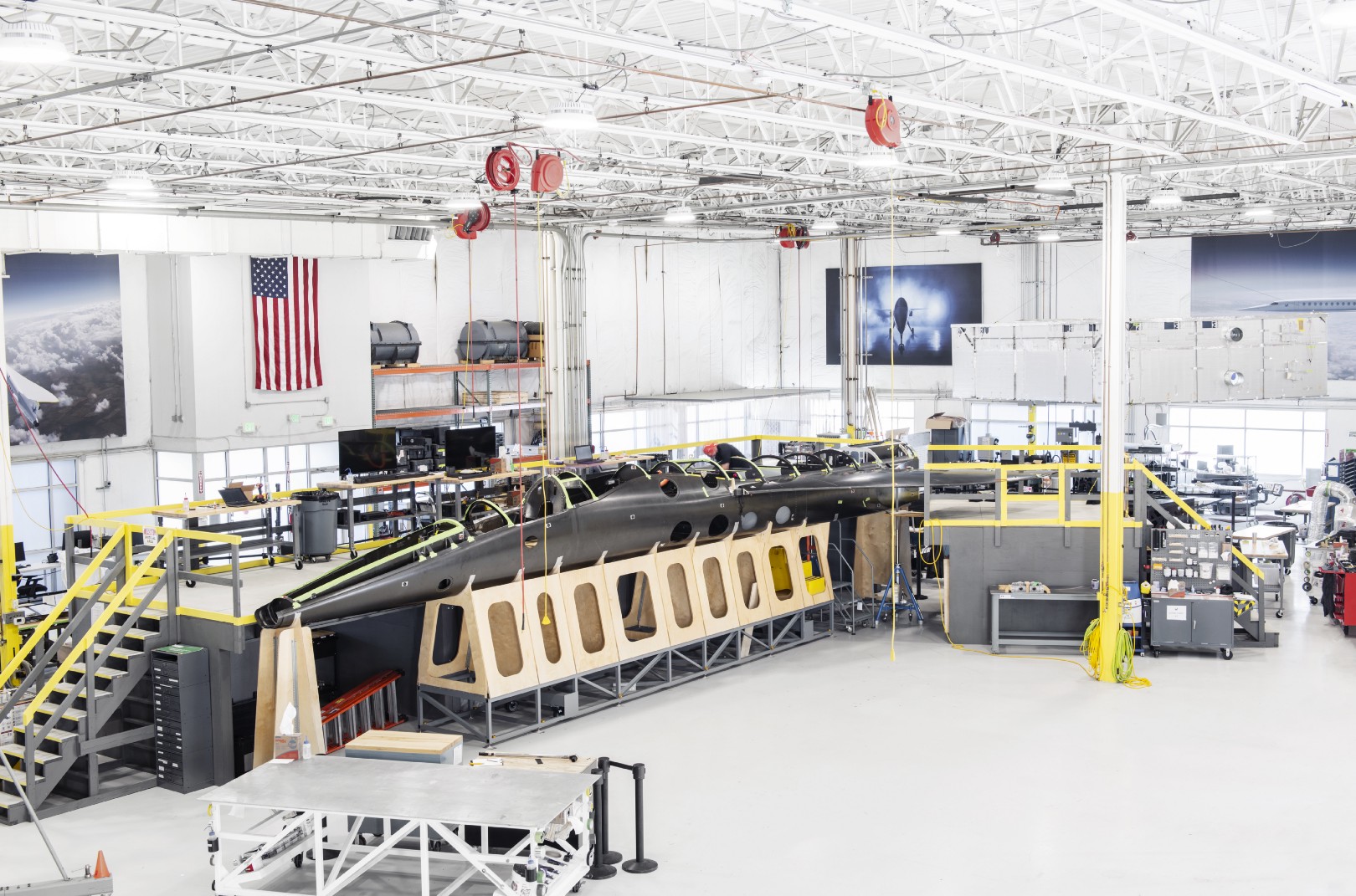
Last month, the company shared a virtual toast to the successful installation of the XB-1 wing structure. Attaching this ogival delta wing to part of the fuselage effectively transformed the aircraft from a simple canoe shape to a nearly assembled jet aircraft. In flight, the wing will provide the aircraft with controllability and stability at both subsonic and supersonic speeds.
Team Leader and Process Engineer Ruslan Pshichenko took the lead in what can be considered the main puzzle: to accurately place the 330 kg wing structure in the ideal position.
“Preparation for this event was aimed at accuracy,” said Pshichenko. "Since this is a very painstaking and demanding process, we first tried on the wing to ensure that everything was aligned as expected."
The fitting, which also served as a dress rehearsal for the final installation, included the active support of 16 team members. Individuals were assigned to specific areas of the aircraft to set the wing in place and control everything, down to the individual spars and clamps.
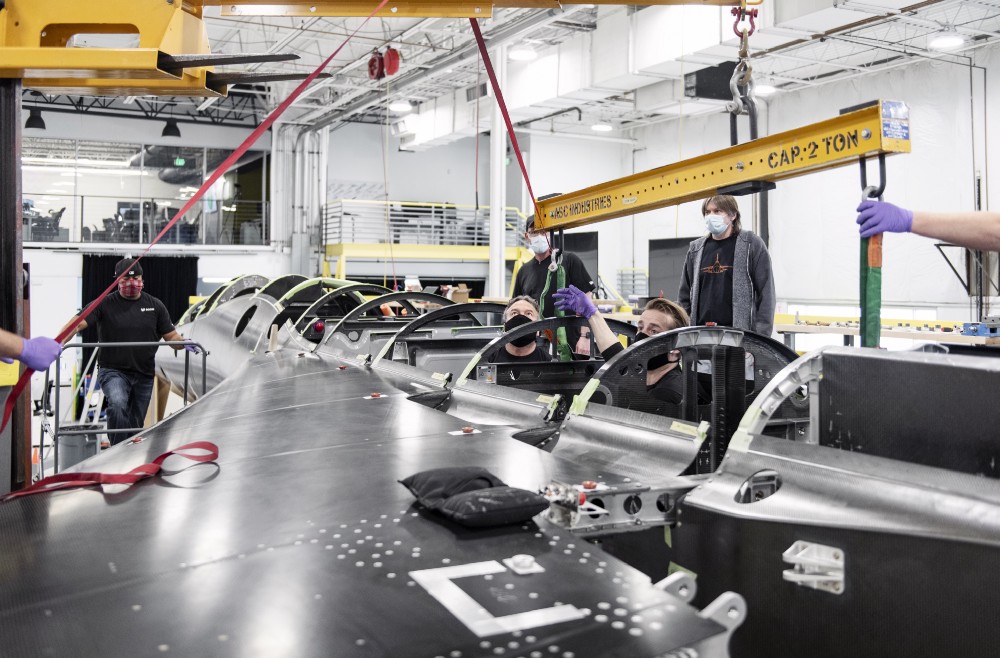
Crew members around the aircraft carefully set the wing structure in place as part of initial training.
“In general, the installation ended with exactly what we needed,” said Pshichenko. "We were able to build an assembly jig for the frame to facilitate reproducibility."
Easy repeatability resulted in quick and hassle-free final installation.
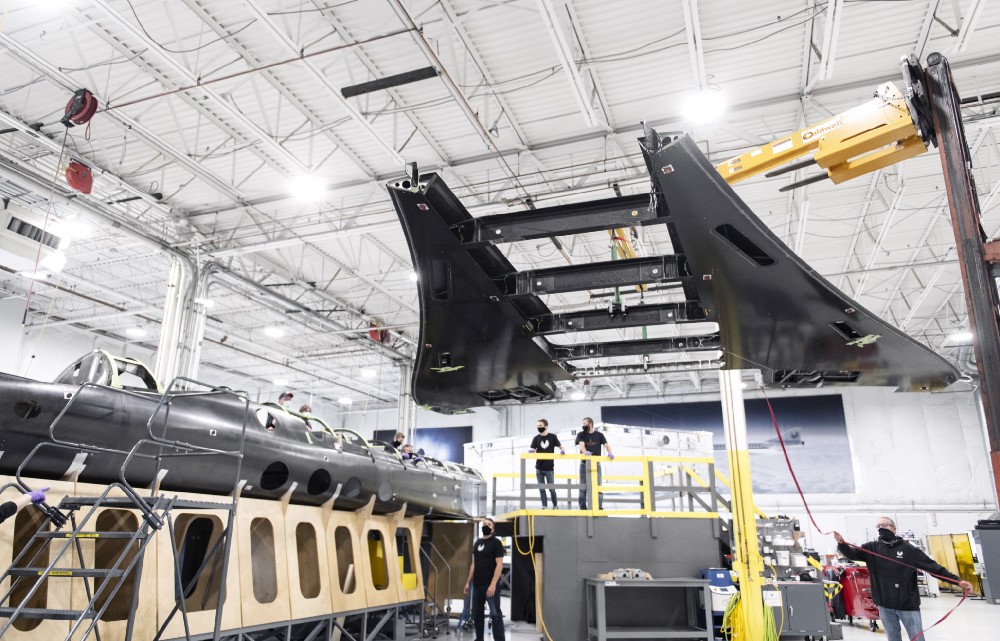
A forklift is used to carefully lift and set the wing structure into place, and the team members on the ground ensure a smooth, safe and smooth process.
“For the official operation, we placed team members on the ground and on a mounting platform to put the wing in place,” said Pshichenko. "We used a forklift to assist the process and have followed the same procedure since assembly." Once everything was in place, the team proceeded to install the final wireframe.
The addition of the wing not only visually transformed the aircraft, but also allowed the team to move on to the next stage of assembly. “Now that this milestone is over, several teams are ready to achieve their own goals,” said Pshichenko. “Designers, avionics, systems and manufacturing groups have come together and can now make further progress. Once completed, the production team installed fuel lines for the four wing spars. "

Successful completion of this phase opened access to key systems and allowed the team to continue building.
While COVID-19 poses undeniable daily challenges, the team has proven an impressive ability to overcome adversity, balance with limited resources, and develop creative solutions.
“We maintain an excellent pace in the hangar, and I am incredibly proud of the team that made this happen,” concluded Pshichenko. "And in the midst of a pandemic, no less."
We print the plane of the future on a 3D printer
( 3D Printing the Future of Flight )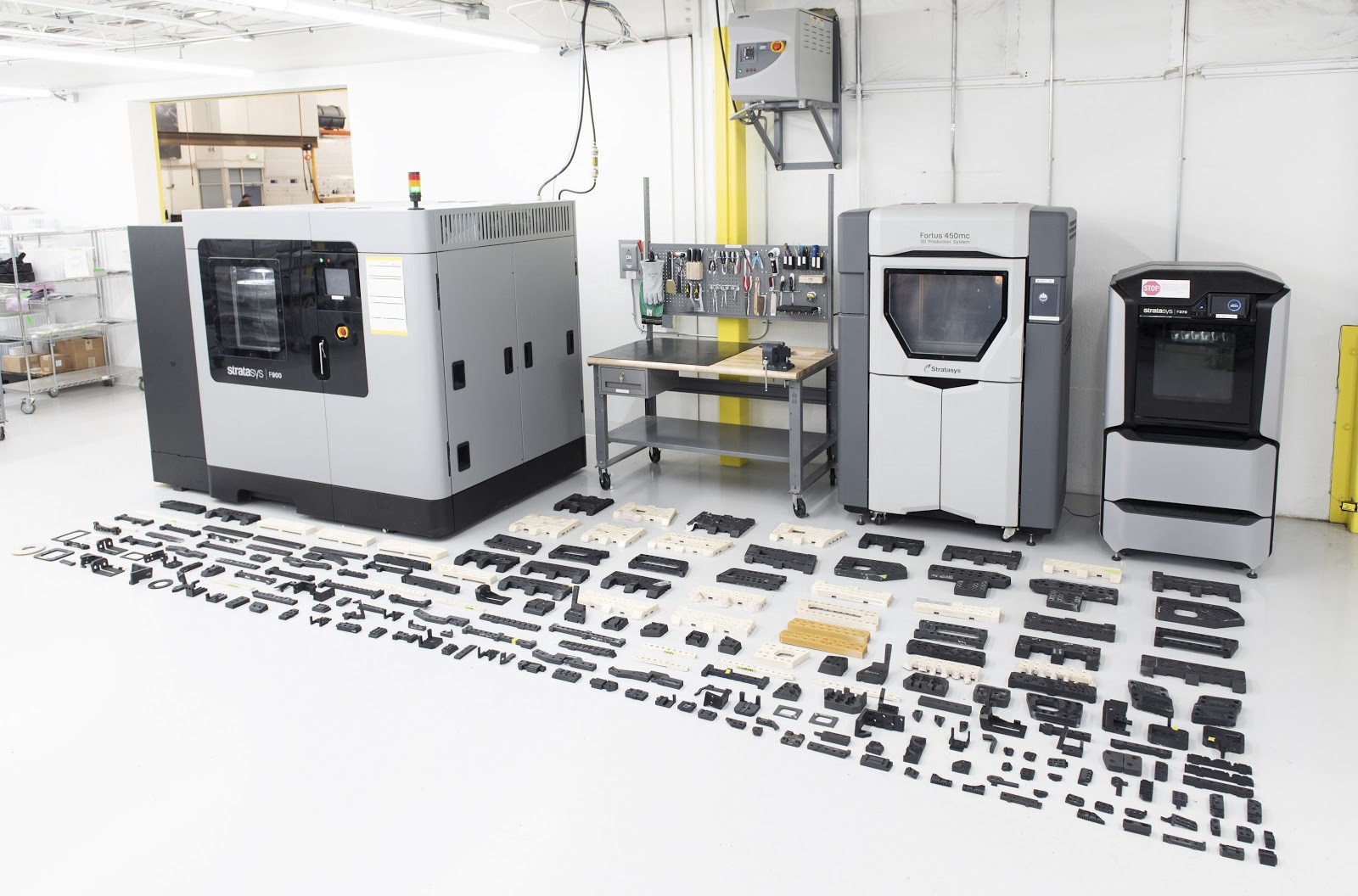
Boom has installed over 300 3D printed parts on its XB-1 supersonic demonstrator.
Powerful motors. Strong metals. Robust chassis.
When we think about airplane parts, we imagine materials and components that are virtually indestructible. 3D printed parts don't come to mind. But advances in materials and 3D printers are accelerating the process of change that makes 3D printing ideal for prototyping, tools, and flight equipment, not to mention spare parts, interiors, and even plumbing fixtures.
3D printing is changing the way we design and manufacture aircraft.
For the team behind the XB-1, the Boom's supersonic demonstrator, 3D printing has proven invaluable at every stage of assembly. More than 300 unique parts have been installed on the aircraft. But 3D printing has brought more to the XB-1 than just producing parts.
Three 3D printers, three needs
Early in the assembly of the XB-1, the Boom team partnered with Stratasys to explore the possibilities of 3D printing, also known as additive manufacturing. The program focused on 3D printing to meet three different needs: functional prototyping, tool support, and on-demand production of flight equipment. Three printers met the build needs: Stratasys F900, 450mc and F370.

Stratasys F900, 450mc and F370
A workhorse, the Stratasys F900 takes center stage in the Boom hangar. The F900 prints on several materials including ULTEM 9085 and ULTEM 9085 CG. Both are flame retardant, high performance resin based thermoplastics with high strength-to-weight ratio, excellent heat resistance and high toughness. The team used 9085 to print drill blocks and 9085 CG for the hundreds of parts already installed on the XB-1. 9085 CG comes with certificates of conformity and also has better traceability and process control than standard material, making it ideal for aircraft parts.
The Stratasys Fortus 450mc can also print on a wide variety of materials. The team developed it to print drill blocks from FDM Nylon 12 CF, an incredibly durable material. FDM Nylon 12 CF is impregnated with carbon fiber making it ideal for printing rigid drill blocks. During the assembly of the XB-1 titanium stern fuselage, the team used hundreds of drill blocks, printing them overnight. This not only made the build faster, but also reduced the team downtime.
The Stratasys F370 typically prints from ASA, an economical and less durable material ideal for rapid prototyping and testing of fitting components. The team printed prototypes with the F370 to burn off the risk of any unexpected collisions (collision of parts or mismatched areas where parts join or touch), as well as for installation on existing flight equipment. Testing the parts using 3D printed parts allowed for improvements in the design, so when the team eventually produced the parts, each one fit like a glove.
Stratasys F900, XB-1. , . ECS (Environmental Control System).
:
During the first phase of building the XB-1, one of the main priorities was to prototype components for flight control systems, including mechanisms and mechanical components. The goal of each prototype was to make sure that the part fits and also works together with other parts. With prototypes, the team could check for defects (mismatched parts to be joined) before investing valuable resources in manufacturing the part.
After printing out several iterations over several hours and finalizing projects, the team sticks to the build on schedule. They also avoided the delays that happen when a part comes from the manufacturer and doesn't fit. By keeping these functions internally, the team kept downtime to a minimum.
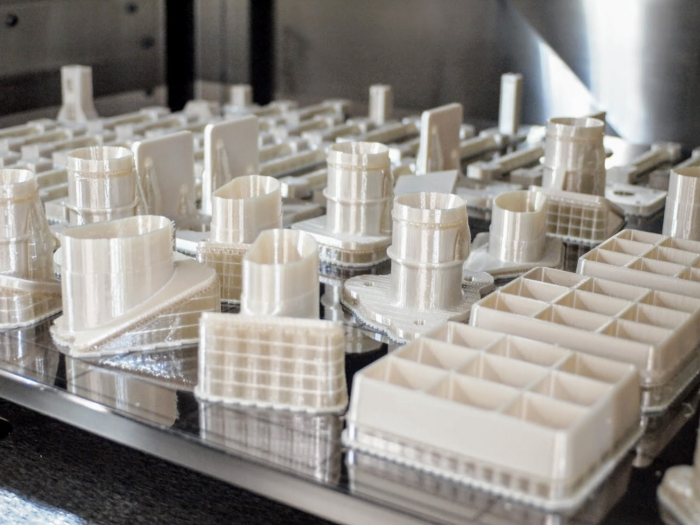
The Stratasys F900 is the workhorse of all production, capable of printing many parts on its massive 914 x 610 x 914 mm print bed.
All printers were involved in prototyping, from the fuel manifold to the engine mounts. The team 3D printed the front engine mounts, for example, to test compatibility with left and right motors. After several iterations, they successfully validated the design during compliance review.
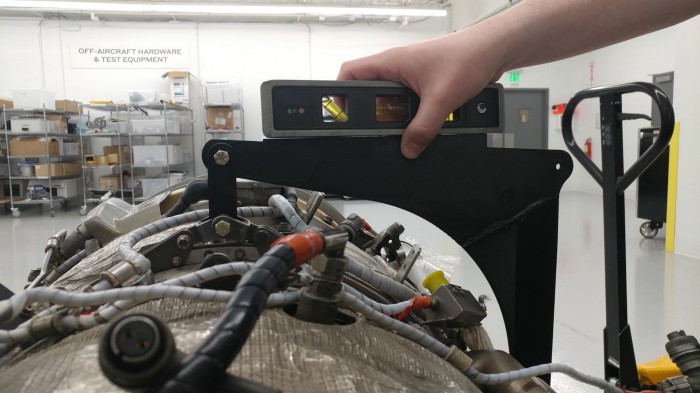
Several 3D printed iterations of this engine mount have confirmed a successful fitting test.
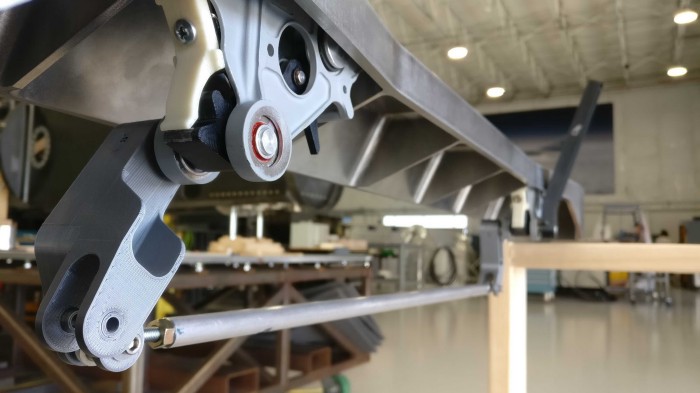
The team 3D printed this prototype bonnet latch mechanism to bring the kinematics up to expectations.
Tools: Improving Accuracy and Reducing Potential Damage
During the assembly of the XB-1, the team leveraged the capabilities of the F900 and 450mc to print over 550 drill blocks. The blocks supported the meticulous assembly of the titanium fuselage along with other printed templates, including for the cockpit bulkhead.
The team used metrology to drill holes in the blocks, resulting in greater accuracy. And with more precision, the team reduced the potential damage to the aircraft.
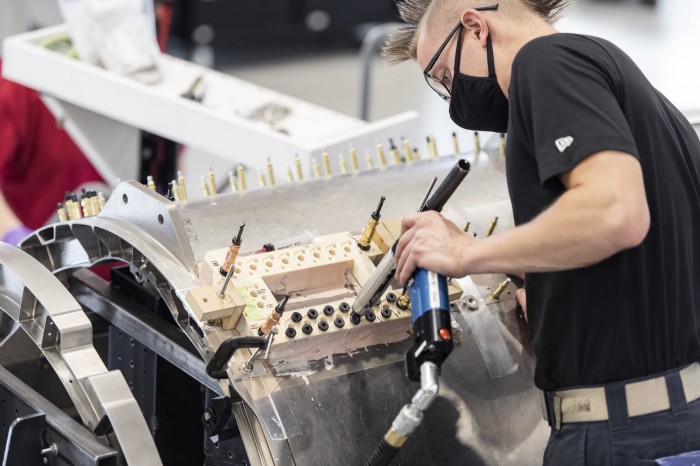
Using 3D printed drilling blocks, the team was able to complete the assembly as scheduled while reducing any potential damage to the titanium aft fuselage.
Without 3D printing, the production time for the drill blocks would be several weeks, not to mention the tens of thousands of dollars that would be required to manufacture from aluminum. Thanks to in-house 3D printing, the same blocks could be printed in a few days at a lower cost.
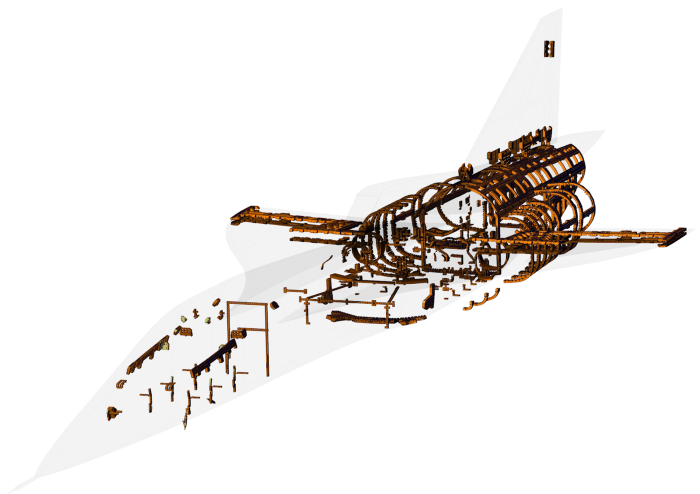
This illustration shows many locations where the team used 3D-printed drill blocks to accurately drill holes.
Metallic materials: 3D printed titanium parts to withstand heat
Thanks to significant advances in the industry, 3D printing is now possible from virtually any material. Silver, photopolymers, stereolithography materials (epoxy resins) and even titanium can be used for 3D printing.
Boom partnered with VELO3D to produce metal parts that would otherwise have taken weeks, if not months. In total, the company 3D printed 21 XB-1 parts, including some of the XB-1's most sophisticated titanium parts: Manifolds for Variable Bleed Valves (VBV), which removes excess air from the engine compressor.
In the case of VBV manifolds, traditional production methods such as machining, welding or casting would be impractical. They were able to achieve the desired geometry of the part only through 3D printing.
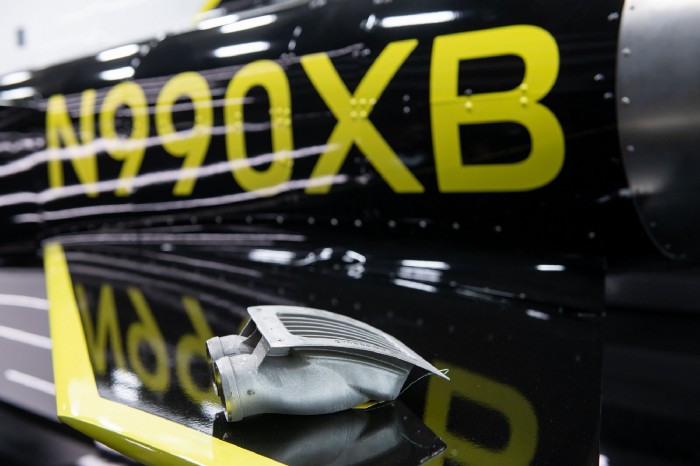
As a result of Boom's partnership with VELO3D, 21 3D-printed metal parts were installed on the XB-1.
Lightweight 3D printed parts: a game changer for aerospace engineers
3D printing not only saved time and resources during assembly, but also reduced the weight of the aircraft, which dramatically changed the rules for all aerospace engineers. Since the weight of an aircraft is directly related to fuel consumption, the goal of aerospace engineering is to create a light aircraft that meets all safety requirements. A lighter aircraft burns less fuel, so any weight reduction is of the essence.
3D-printed parts, depending on the choice of materials, can be significantly lighter than their traditional steel and aluminum counterparts. On the XB-1, which has over 340 unique 3D printed parts, the overall weight was of the essence.
Now that the production group has handed the XB-1 over to the ground and flight test team, they are turning their attention to the design and construction of the future Boom supersonic airliner.
And for Overture, the possibilities for 3D printing seem endless, with the ability to 3D print the interior of the cockpit, flight control panel, and galley - in addition to prototyping, refueling and flight equipment.
Advances in 3D printing, fueling these capabilities, will open up new opportunities for lower manufacturing costs, faster production times, and lower emissions through lighter aircraft.
Parts printed on a 3D printer
( Boom's 3D Printed Aircraft Parts Reveal the Future of Manufacturing )3D printing technology is taking the manufacturing world by storm. From consumer product design to medical modeling, a growing number of companies are realizing the value of 3D printing for rapid prototyping and manufacturing.
Perhaps no industry has seen such a major impact as the aerospace industry. Boeing is projected to save up to $ 3 million on each aircraft by 3D printing titanium parts on the 787 Dreamliner. Honeywell has saved over seven months in redesign through additive manufacturing. Airbus has managed to create spacer panels for overhead storage 15 percent lighter than previously possible.
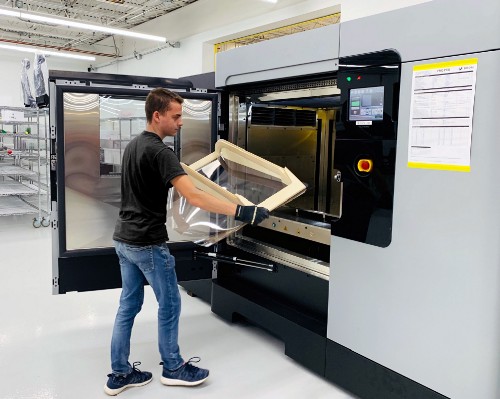
Boom began using 3D printing more than two years ago as part of a partnership with global leader Stratasys. Since then, the company has produced hundreds of 3D printed parts, tools and prototypes and saved thousands of hours of labor time. Stratasys and Boom recently announced a seven-year extension to 2026 and will continue to deliver creative solutions to complex problems.
The benefits for Boom and many similar companies are enormous. The three most notable benefits include savings in time, money and weight. Many aircraft parts are inherently complex geometries, in part due to lack of space and weight. Before 3D printing, complex parts were milled from a single block of material, which often became extremely costly, painstaking, and time-consuming.
How effective is 3D printing for Boom? We share five unique printed components that are shedding light on the future of aircraft manufacturing:
Brake pressure regulator
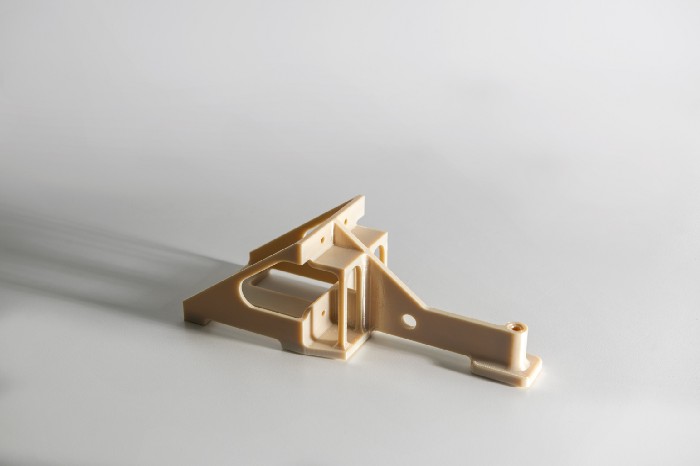
The brake pressure regulator is used to mount the strut pressure control components in the A-pillar hydraulics. If this part were made of aluminum, as is traditionally done, it would take over 6 weeks and $ 2,000. This flight prototype took only 9.5 hours to print and cost $ 70 for materials.
Mount for telemetry connection
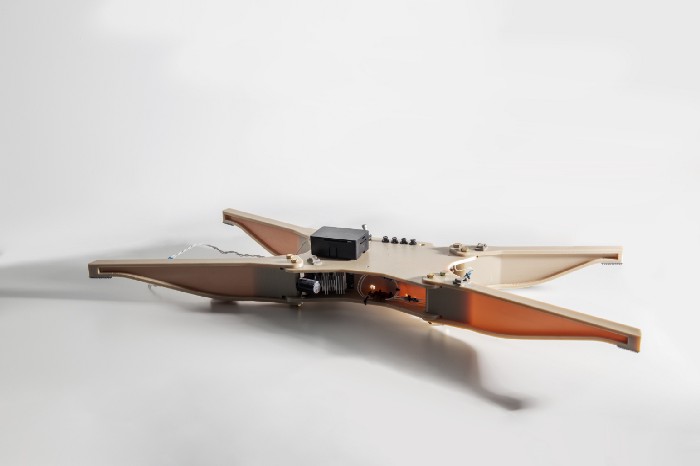
This part served as an equipment mount when testing the telemetry connection. As part of the tests, a team of engineers installed equipment at Pikes Peak, 4,300m in Colorado, and confirmed that reliable telemetry connections between the aircraft and the ground station could be maintained up to 200 miles.
Flight control system test rig
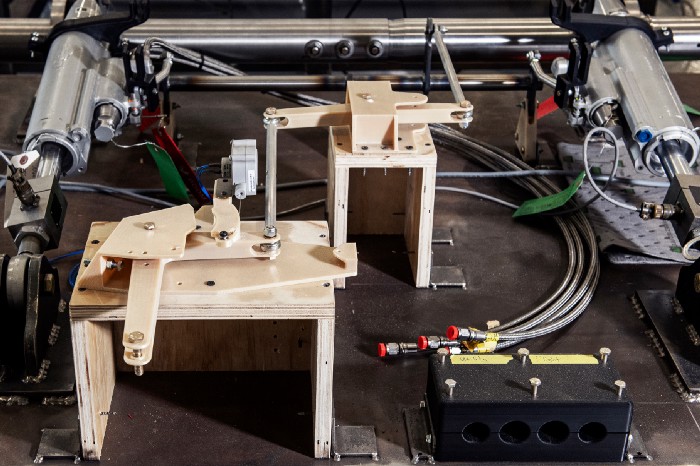
Used to validate the deflection mechanics of tail drives, this flight control test fixture allowed rapid and thorough safety testing at very low cost. Although this printed part will not be used for flight equipment, it has enabled engineers to ensure that the flight equipment actuators work properly.
Compressor bleed air duct
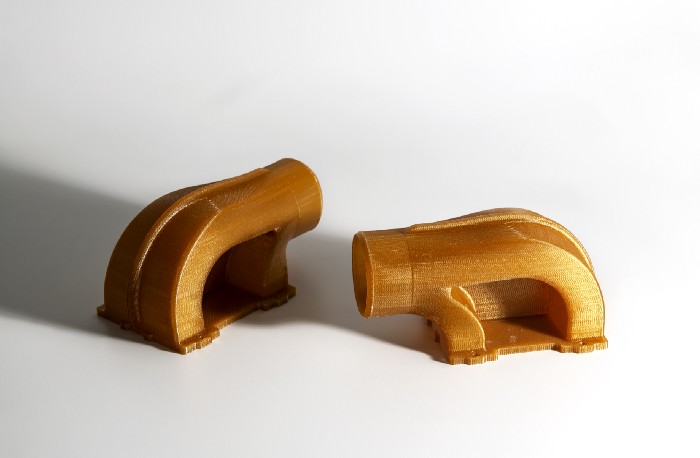
Another great example of rapid prototyping, this compressor bleed duct was used during engine testing to reroute air from the engine's internal circuit and as a test piece to ensure a final fit during flight. Without 3D printing, such parts would likely be devoid of functionality, a process that requires many different parts to be used to get the most efficient shape.
A traditional aluminum part would be significantly more complex to design and cost roughly 4 weeks and $ 4000. For Boom, this part only took 14 hours and $ 150.
Flight equipment pallet
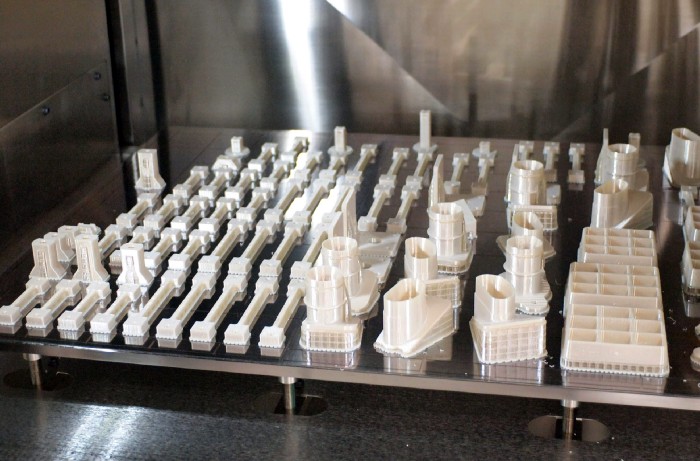
This 94 hour print job of over 70 parts was completed on a Stratasys F900 Flight Equipment Pallet for various aircraft systems. With the benefits of electrical, wing, flight controls, hydraulics, and fuselage, this single job saved thousands of dollars and weeks of lead time over traditional manufacturing methods.
We are grateful to Dmitry Kudryavtsev and Varya Sheremet for their help with translation.
On November 9, 2020, the free Startup School for Future Founders by Y Combinator has started from the world's best accelerator, and we will publish useful translations for those who plan to become a founder of an international startup.
follow the newsYC Startup Library in Russian on the telegram channel or on Facebook .
Useful materials
- Y Combinator: Russian-speaking founders
- What startups Y Combinator is looking for in 2020
- A selection of 143 translations of Paul Graham's essays (from 184)
- All 16 lectures of the Y Combinator startup school with Russian subtitles of the "Spring 2017" version
- All 20 lectures of the Y Combinator 2018 startup school with Russian subtitles
- All 21 lectures of the Y Combinator startup school with Russian subtitles 2019
- Chat Y Combinator in Russian
- Public on Facebook YCombinator in Russian