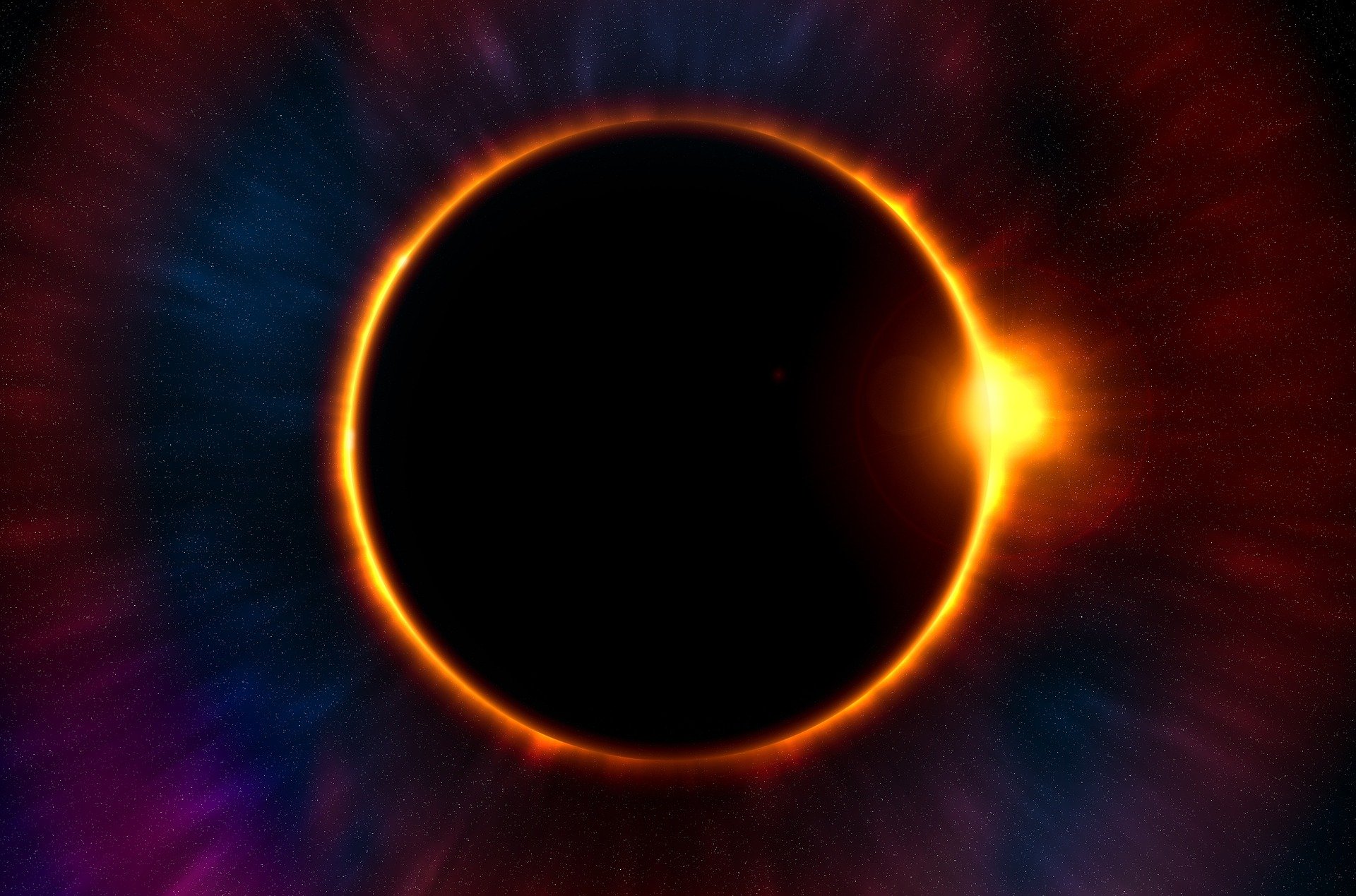
We have already written about unexpected and remarkable ideas and developments in the field of obtaining energy from nuclear fission. And also about what to do when something goes wrong with nuclear reactors. As we know, freedom is better than non-freedom, and synthesis is better than decay. This is exactly what scientists thought a hundred years ago, when they took the first steps to tame thermonuclear fusion. In this article, we will briefly describe what thermonuclear fusion is, at what stage are scientific developments and when it is worth waiting for the introduction of a new method of energy production. After all, this is precisely why mankind needs him.
Staring at the Sun: the history of the discovery of thermonuclear fusion
With the development of science, mankind began to wonder how the Sun works, why it does not go out and continues to emit heat and light. Back in the twenties of the last century - almost a hundred years ago - British scientist Arthur Stanley Eddington came up with the ideas of the proton-proton cycle, that is, a set of thermonuclear reactions, during which hydrogen in stars turns into helium. And this reaction is accompanied by the release of colossal amounts of energy, which can be easily felt by simply going outside on a sunny day.
A little later, already in the thirties, scientists from the University of Cambridge, led by Australian Mark Olyphant, as a result of a series of experiments, discovered nucleons (the common name for the protons and neutrons that make up the atomic nucleus) of helium-3 and tritium, which take part in these reactions, and their German counterpart, Hans Bethe, received the Nobel Prize in Physics for his contributions to the theory of nuclear reactions and, especially, for his discoveries concerning the sources of energy in stars. Already in 1946, Sir George Padget Thomson and Moses Blackman described and patented the idea of the Z-pinch, that is, a plasma confinement system using a magnetic field or "magnetic trap", which formed the basis for further experiments to create the first devices for controlled thermonuclear fusion.
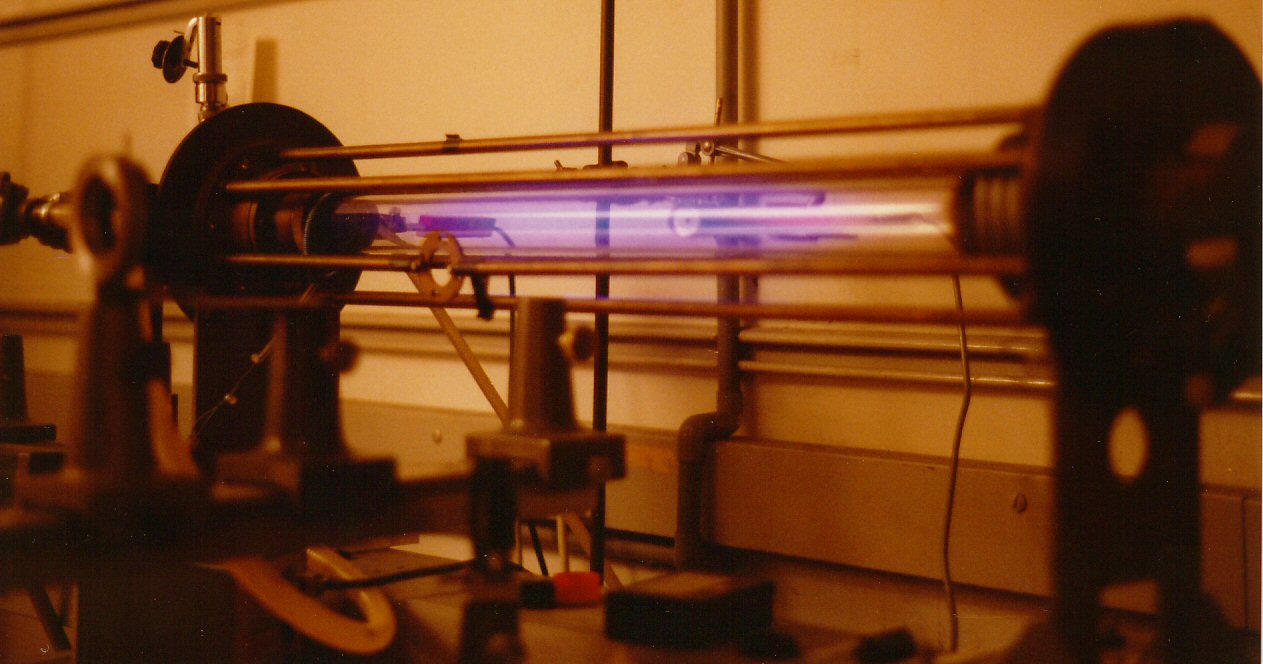
Laboratory magnetic trap, photo: Sandpiper / Wikimedia Commons
Infinite Power: Advantages, Disadvantages, and Obstacles to Implementation
Let's move on from history to general theory. Controlled thermonuclear fusion is the process of obtaining heavier atomic nuclei from lighter ones with the goal (in theory) of using the released energy to generate electricity. In essence, it is the opposite of the fission reaction used in traditional nuclear power. Basically, deuterium and tritium are used for the thermonuclear fusion reaction (the so-called DT reaction), although variants with deuterium and helium-3 are also possible, between the nuclei of deuterium (DD) and other combinations of isotopes.
By themselves, atomic nuclei do not interact very willingly because of the "Coulomb barrier", that is, the forces of electrostatic repulsion between them. To overcome it and start a reaction in terrestrial conditions, the substance must be heated to a sufficiently high temperature, and in this case we are talking about hundreds of millions of degrees. It is from this process that thermonuclear fusion got its name. The combination of deuterium and tritium in this case requires a "minimum" temperature for the start of the reaction (those same 100 million degrees), so it is most often used in experimental installations.

DT fusion reaction. Source: Toshiba Energy Systems & Solutions Corporation
Also, in the course of the reaction, a large number of neutrons appear, but we will talk about their meaning a little below, and first we will try to explain why the commercial application of this process has generally excited the minds of mankind for the last 70 years. So, the advantages of controlled thermonuclear fusion:
- Comparative availability of isotopes for the reaction. Deuterium can be easily obtained from seawater, the reserves of which are more than sufficient on Earth. Tritium does not occur in nature, since it has a half-life of only 12.3 years, but it is obtained from lithium-6 and heavy water from nuclear reactors, which we are not ready to abandon in the coming years.
- — , , 1 34 , — 44 . 17,6 (), 170 1 .
- - «» , , — , , .
- , . . , , , .
In addition, during thermonuclear fusion, substances are not released that can later be used to make "dirty" weapons.

Tokamak JET, photo: EFDA JET / Wikimedia Commons
But why, then, the very principle of controlled thermonuclear fusion, developed in the middle of the last century, has not yet been implemented in practice or implemented only as experimental installations that have not begun to produce electricity? Let's look at the disadvantages and limitations of this process.
Let's go back to our neutrons first. During the reaction with the use of DT, a neutron flux is formed, which bombards the walls of the containment of the reactor. As a result, we are dealing with the so-called "induced" radiation, which greatly complicates the maintenance of the equipment and, quite possibly, will lead to the need for its periodic replacement, since over time, from neutron bombardment, materials become not only radioactive, but also fragile. To solve this problem, it is proposed to use materials that are not sensitive to radiation, which will last longer, but their use will increase the already colossal costs of building thermonuclear fusion power plants. The use of other active substances is also considered in order to obtain "neutron-free" reactions,but we have already discussed the requirements for density and reaction temperature for them above.
Even at the current level of technology development, scientists and engineers cannot ensure that the energy consumption for heating and bringing the substance in the reactor to a plasma state, and then maintaining it in this state, despite the constant loss of heat (as well as for cooling the system, work electromagnets and other subsystems), fell lower than the amount of energy released during the reaction. For example, the British JET tokamak has reached a ratio between the incoming and outgoing energy of only 67%, that is, 0.67 Q. Q is an indicator that expresses the ratio of the amounts of energy expended and received in such a system, and so that the fusion reaction is considered self-sustaining , it must be equal to at least 5, and much higher to generate useful capacities. Today, there are no reactors with such a value in the world.
The final question, of course, is payback and cost. To achieve an accurate imitation of the reactions inside the Sun, it is not enough just to take tritium and deuterium and bring a conditional match to them. A thermonuclear fusion reactor is an incredibly complex, bulky and expensive design, which has a place for a massive cooling system, a huge number of electromagnets of various types, and even its own power plants.
It is estimated that the construction costs of the ITER experimental tokamak (see below), which has not yet been completed, could exceed $ 20 billion. At the same time, the reactor is generally not designed to generate electricity, that is, the only profit from the operation of ITER will be the experience of joint work of scientists and experimental data.
Practical magic: the main types of construction and milestones of their development
Conventionally, installations for controlled thermonuclear fusion can be divided into four types: tokamaks, stellarators, mirror traps, and pulse systems. Using their example, we propose to consider both the development of ideas that in the future can lead to the production of electricity using thermonuclear fusion, and "dead-end" branches, which, for one reason or another, in the coming years (or never) will not go beyond the framework of theory and experiments. ...
TokamakIs an abbreviation for "toroidal chamber with magnetic coils", which chamber is the main element of the reactor, which serves to contain the plasma. In this case, magnetic coils wound around the reactor chamber are used in order to create a special field that keeps the plasma from contacting its walls, which modern heat-insulating materials simply could not withstand. At the same time, a current is also passed through the plasma itself, which serves both to heat it and to create a poloidal magnetic field. In modern conditions, this field cannot exist for more than a few seconds, and without it, the plasma loses its stability, therefore, it is too early to talk about the use of tokamaks for continuous production of electricity.although it is possible to maintain the current for a longer time using microwave radiation or injecting neutral deuterium / tritium atoms into the plasma.
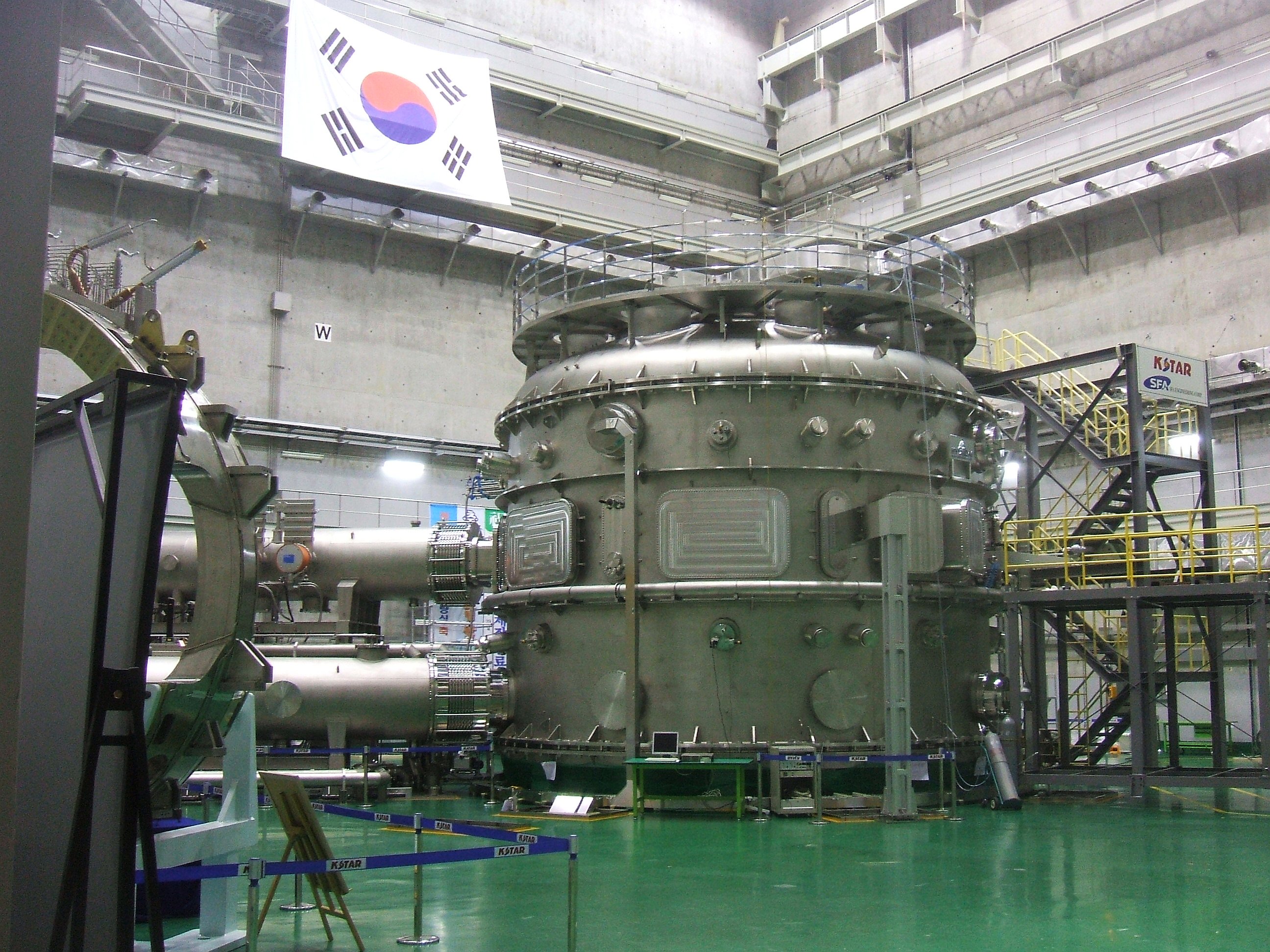
Tokamak KSTAR, South Korea, photo: Michel Maccagnan / Wikimedia Commons
Tokamak ideas were first described in the Soviet Union back in the 50s of the last century, and the first such reactor was built at the Kurchatov Institute in 1954. For a long time, tokamaks remained a purely Soviet development, but in the 1970s British scientists confirmed the record results of plasma heating achieved at the Soviet T-3 tokamak, and became interested in the technology around the world.
Today tokamaks are considered the most promising development, and their number in the world exceeds the number of other types of installations. Among the achievements in this area, it is worth noting the Chinese EAST (Experimental Advanced Superconducting Tokamak, built with the support of the Russian Federation), which reached a plasma temperature of 100 million degrees in 2018, the European JET (Joint European Toru), which is located in the UK and is considered the largest tokamak in world, as well as the ITER already mentioned above, which we will dwell on in more detail.
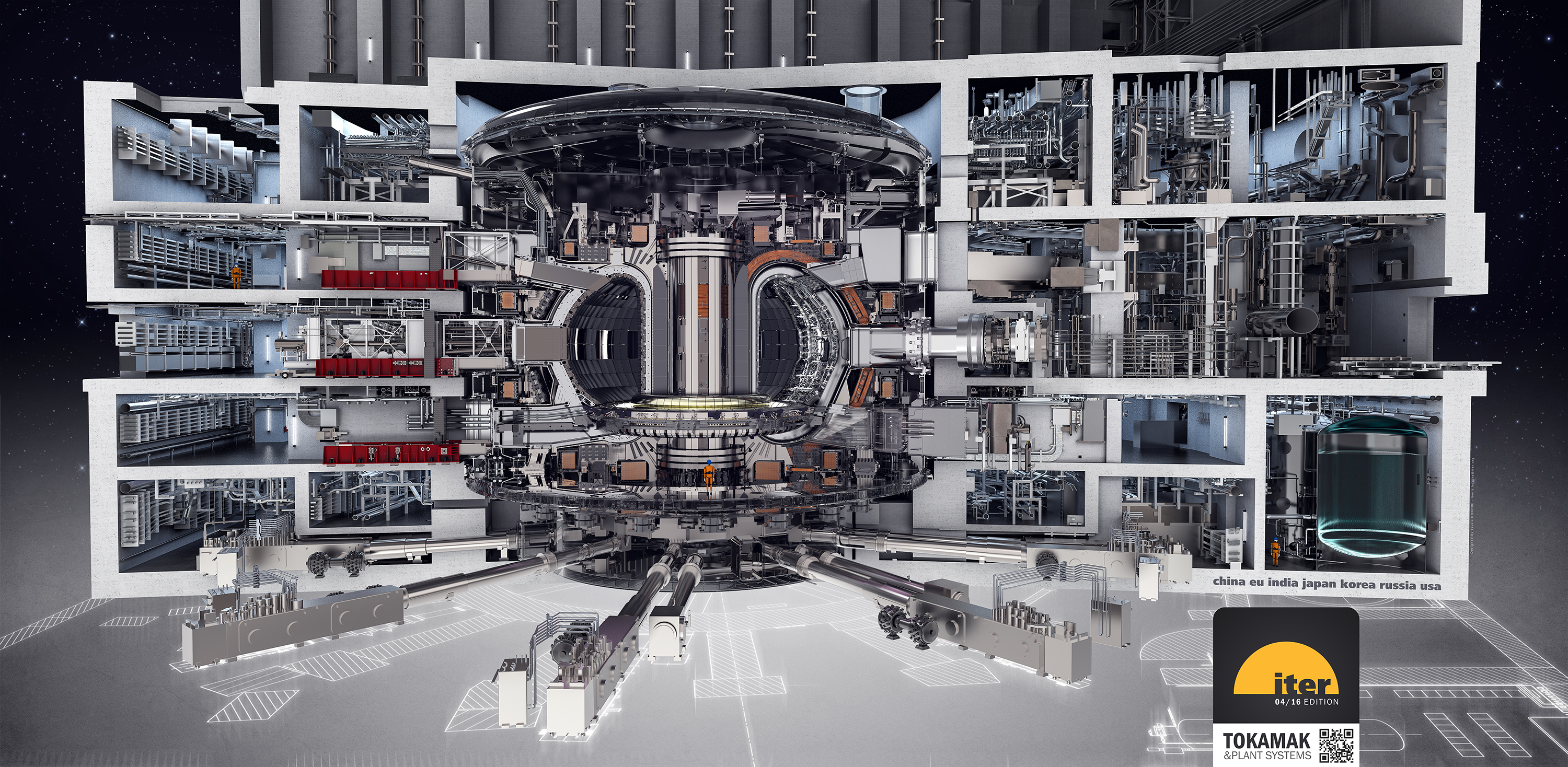
ITER tokamak circuit. Source: Oak Ridge National Laboratory - ITER Tokamak and Plant Systems (2016) / Wikimedia Commons
The idea of building an ITER (International Thermonuclear Experimental Reactor, international thermonuclear experimental reactor) was discussed back in 1985, at a meeting between Ronald Reagan and Mikhail Gorbachev, but the real construction began only in 2010. Many countries are involved in the work on the reactor, including Japan, the EU, Russia, the USA, South Korea, China and India. The result of the joint project will be a giant structure weighing 23,000 tons, which will displace JET from the pedestal of the largest tokamak on the planet and, in theory, will be able to bring the Q value to 30.although the creators of ITER do not set themselves the goal of achieving electricity generation - the task of the tokamak is to finally prove the very possibility of using thermonuclear fusion in this area and to pave the "way" (this is how the abbreviated name of the reactor is translated from Latin) for DEMO, the first tokamak with a "positive" balance, which will not start until the middle of the 21st century.
In the ITER project, Japan was responsible for the development and production of one of the most important elements - superconducting coils required to form a magnetic field around the reactor chamber. Specifically, Toshiba is developing a giant 16.5-meter toroidal field coil that weighs around 300 tons. At the same time, it is necessary to observe extremely strict tolerances for the dimensions of each part - only a few millimeters - so the technologies and methods invented during the work on the Japanese experimental tokamaks, the JT-60 and JT-60SA, become of great help.
Stellarators(from lat. stella - "star") got their name because of the similarity of the processes in the reactor with those that occur inside the stars. The first prototype was built in 1951 in the United States under the leadership of its inventor, Lyman Spitzer. The main difference between stellarators and tokamaks lies in the design of the magnetic trap: in stellarators, only external coils are used to confine plasma in the chamber, which create lines of force rotating around the chamber. This design theoretically allows the use of a magnetic trap in a continuous mode. In stellarators, as in tokamaks, a mixture of deuterium and tritium is almost always used, which is introduced into the vacuum vessel of the chamber. Modern designs have abandoned the torus-shaped chamber in favor of complex computer-simulated models.Their goal is to maximize plasma containment efficiency.
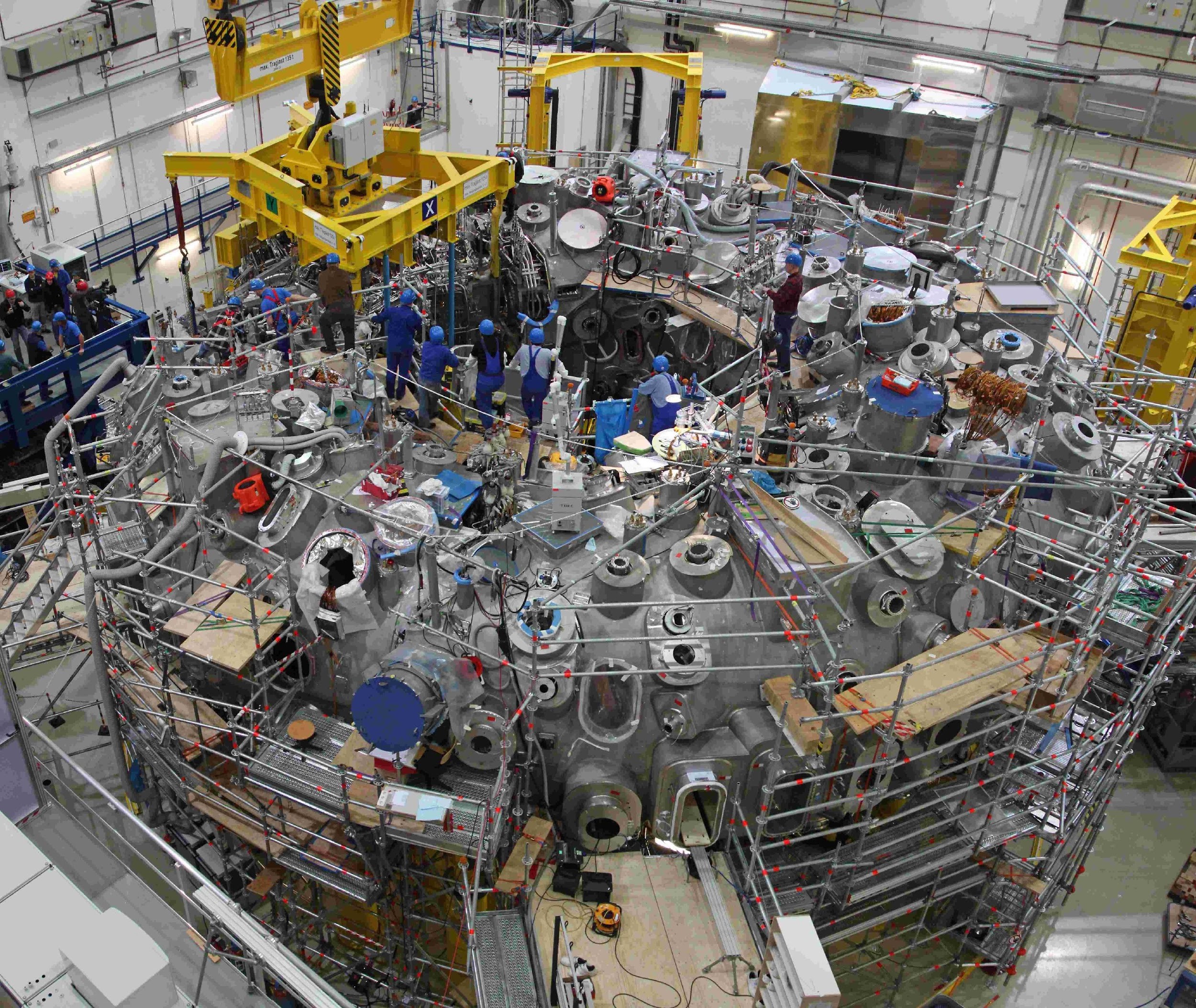
Wendelstein 7-X. : Max-Planck-Institut für Plasmaphysik, Tino Schulz / Wikimedia Commons
Despite the possibility of continuous exposure to plasma and the modified design of the chamber, stellarators are not as widespread as tokamaks. This is primarily due to the greater complexity of the design and their lower efficiency in modern conditions. The Wendelstein 7-X, built in Greifswald in Germany in 2015, became the largest stellarator in the world and a kind of "epitaph" for this development. According to the calculations of scientists, he had to bring the time of continuous exposure of electromagnets to plasma to 30 minutes in order to demonstrate the possibility of using stellarators for long-term generation of electricity. At the same time, in 2018, during the experiment, the plasma temperature was only raised to 40,000 degrees Celsius, and the operating time was brought to 100 seconds. The next tests are scheduled for 2021.
- this type of controlled thermonuclear fusion plant remains largely a theoretical development. Even Academician Andrei Sakharov in 1960 proved that thermonuclear fusion is possible without the use of magnetic traps, proposing the opposite of the classical approach. In this case, we are not talking about a super-rarefied plasma, which is held in place by electromagnetic fields for a long time, but about its superdense (and extremely short-lived) version. It is proposed to detonate miniature "targets" with a frozen DT composition in pulsed systems using powerful lasers or radiation beams in order to achieve a kind of analogue of fuel explosions in gasoline engines, only at the level of thermonuclear reactions. Such a system with periodic explosions can provide an almost continuous chain of thermonuclear reactions that generate energy,while (in theory) without damaging the reactor shell.
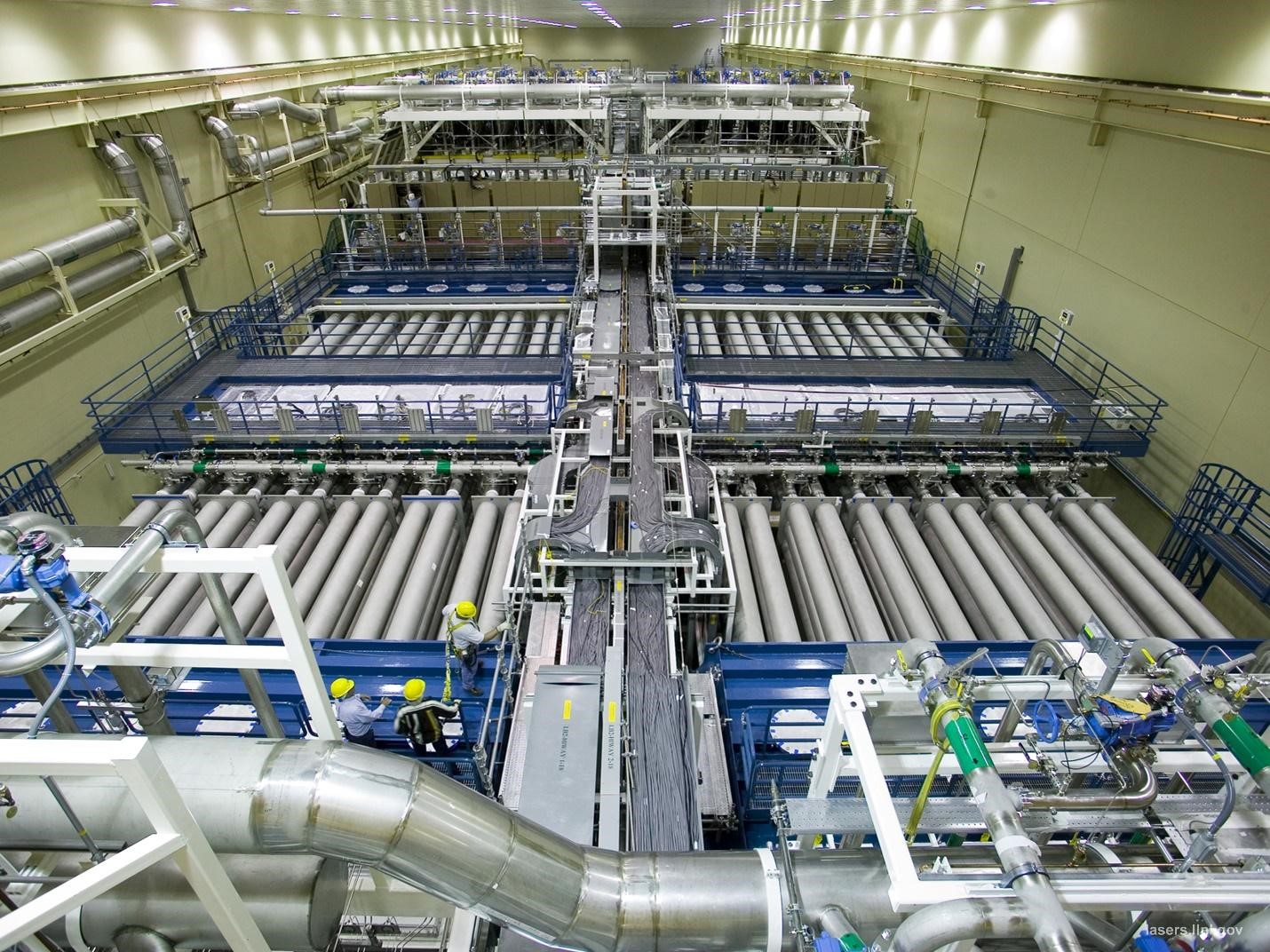
NIF/ : Lawrence Livermore National Laboratory, Lawrence Livermore National Security, LLC, and the Department of Energy — National Ignition Facility / Wikimedia Commons
Among the existing developments in this area, it is worth mentioning the MagLIF project and the NIF (National Ignition Facility, or National Complex for Laser Thermonuclear Reactions) of Lawrence Livermore National Laboratory in California. Despite the continuing potential of this idea, in 2012 the US government planned to end funding for the program due to scanty practical results. As of today, experiments continue, but the complexity of the "targets" themselves and the need for regular delivery of them to the chamber, in which an explosion, equivalent to a ton of TNT, then occurs, leave this type of installations far behind tokamaks and stellarators in terms of practicality.
Mirror traps- The first experiment using "open" magnetic traps was carried out back in 1955 in the same Lawrence Livermore National Laboratory. The idea behind the traps was to use not a closed torus, but an elongated magnetic vessel, open at two opposite ends. In this case, the "new" plasma had to heat up to the required temperature, give off energy and exit through the side holes (or be bounced back by the magnetic field, as from mirrors - hence the name). Thanks to this form and mechanism, their cost was much lower than that of competing designs, so for a while mirror traps seemed to be an extremely promising development. But over time, experimenters faced plasma instability, poorly understood at the time of the start of development,which led to problems and the impossibility of reaching the temperatures required for thermonuclear fusion. Subsequently, the design was repeatedly changed, but the ambitious American MFTF installation, for example, was closed even before the start of test runs, since the tokamaks were ultimately simpler, more powerful and cheaper.
Of the interesting developments of this type, it is worth noting the Russian GDL (gas-dynamic trap) from Novosibirsk, which is being created on the basis of the Soviet project of the 50s, the "open" trap "Budker's mirror cell". As of 2018, scientists from the Novosibirsk Institute of Nuclear Physics of the SB RAS managed to reach a temperature of 10 million degrees, and in 2020 they received a grant from the Ministry of Education and Science of the Russian Federation for the purchase of new equipment to continue experiments.
Beautiful tomorrow: instead of conclusions
Among the scientists dealing with the problems of thermonuclear fusion, there is a joking saying that “there are only 30 years left before the success of research and the start of commercial use of reactors,” and they have answered this way for more than a dozen years (stability!). Nevertheless, technologies will continue to develop, and humanity will look for ways to "tame" thermonuclear fusion and create a miniature artificial Sun that will provide our electricity needs without the risk of repeating the Chernobyl disaster and without permanent harm to the planet's ecology. Developments such as ITER may have a direct impact on this research, and we are delighted that Japan and Toshiba are directly involved. And what will happen next ... we'll see in 30 years.