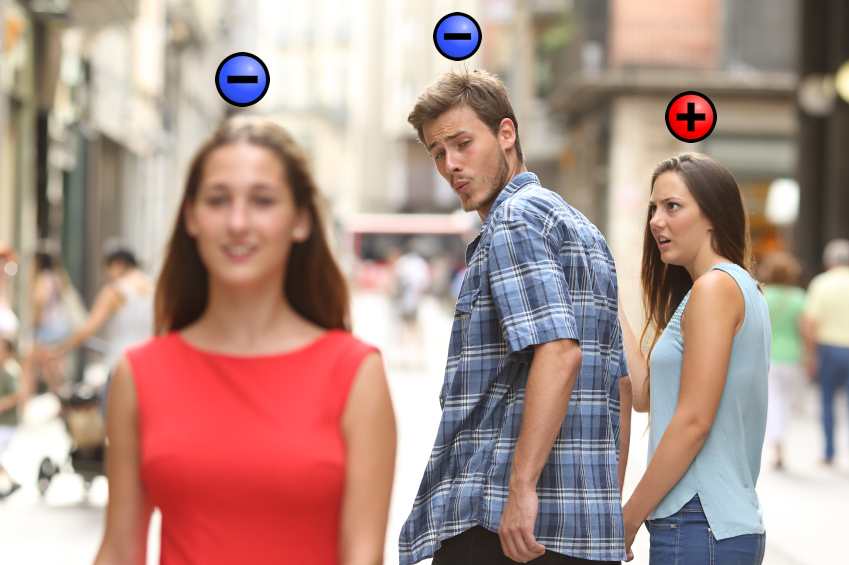
Opposites attract. This worldly principle concerning relations between people does not always correspond to reality. But in physics everything is as they say: opposite electric charges, for example, always attract, and similar ones repel. This principle is as old as the world itself, but it can also be subjected to some modification if other physical laws and phenomena are applied. A group of scientists from the University of Southampton (UK) conducted a study in which they managed to create a new type of material called a photon-bound exciton. The most relish is that photons became a connecting link between negatively charged electrons, which, according to logic, should have been repelled. How exactly were the photons used, what are the features of the invented atom,and in what areas can this development be used? We learn about this from the report of scientists. Go.
Basis of research
As we have already remembered, like charges (i.e. identical: ++ or - -) should repel each other, and opposite charges (i.e. opposite ones: + - / - +) should attract. However, the picture of this interaction changes if you add a pinch of photons, i.e. particles of light. In this case, the influence of the photoelectric effect is added - the interaction of light and matter, when the energy of photons is transferred to matter.
In this work, scientists have created a nanodevice that captures electrons into nanoscale quantum wells *... If the photons bring a lot of energy into the device, then this leads to the exit of electrons from the well. By placing this device between two gold mirrors, you can trap the photons. This will focus the energy of the photons on the electrons, enhancing the interaction between light and matter. The addition of mirrors caused the negatively charged electrons to remain in the well (without mirrors, photons expelled them from the well) and began to bind to each other.
Quantum well * is a potential well that limits the mobility of particles from three to two dimensions (i.e., particles begin to move in a flat layer).The most important role in the performance of the entire system is naturally played by the above-described quantum wells (QW from quantum well ). There are several reasons for this, scientists say.
Firstly, QW allows you to achieve a greater strength of the connection between light and matter, which can be regulated by changing the electron density * in QW.
Electron density * - in quantum mechanics, a measure of the probability that an electron will occupy an infinitely small element of space surrounding any conventional point.Second, the quantum wells can be made narrow enough to obtain one localized electronic subband, which will not have any intersubband transitions.
Third, in such a system, the Coulomb interaction does not create bound states.
From the last two points it follows that pure quantum wells without an surrounding photonic resonator do not represent any discrete resonance at all, but only a continuous absorption band at frequencies exceeding the ionization threshold.
The absence of the Coulomb interaction is justified by the quasi-parallel dispersion of the two electron subbands, which leads to a repulsive electron-hole interaction * .
Electron-hole interaction * (pn interaction) - the area of contact of two particles with different types of conductivity - hole (p from positive - positive) and electronic (n from negative - negative).This is very different from the cases of interband transitions at shorter wavelengths, where the electron-hole interaction is attractive and leads to the creation of narrow resonances outside the electron-hole continuum in the absence of polariton effects.
Thus, the formation of polaritons * can change existing resonances, but does not lead to the creation of new localized electron resonances.
* — , ( , , , ..).

№1: . 1 — , (EX) (EG) - ; 1b — - , ; 1 — , , (EI); 1d- the initially filled subband of electrons has a positive effective mass, and electron-hole mapping leads to a positively charged hole with a negative effective mass.
The images above are a schematic of the above phenomenon. In the case of interband transitions in undoped quantum wells, the electrons participating in the transition initially occupy the valence band with a negative effective mass. However, in the case of intersubband transitions in doped quantum wells, the same role is played by the first partially filled conduction subband, which has a positive effective mass * . In conventional electron-hole mapping, this results in a positively charged hole with negative effective mass.
* — , .The effective mass of electrons in the excited subband m 2 in GaAs quantum wells is greater than the mass in the first subband m 1 . This leads to a negatively reduced mass of the intersubband electron-hole pair m r -1 = m 2 -1 - m 1 -1 .
In the presence of any attractive potential of two bodies, negative mass leads to a repulsive electron-hole interaction, which, in turn, cannot create bound states.
For practical confirmation of the presence of photon-mediated bound states, a system was created consisting of 13 GaAs / AlGaAs quantum wells embedded in narrow grating gold microcavity resonators.

Image No. 2: diagram of the experimental setup. 2 - distribution of the electric field component orthogonal to the metal layers for one period (D) of the structure and for the TM02 mode of the ribbon resonator; 2b - microscopy of a set of samples; 2c - experimental setup used to measure reflectance (mid-infrared microscope connected to a FTIR spectroscope.
The resonators are one-dimensional ribbons, and the electromagnetic field ( 2a circuit ) is almost completely contained under the metal pins.
The dimensions of the quantum wells were thin enough to have only one trapped conduction subband, since the presence of the second subband would lead to the creation of intersubband polaritons.
If there were two subzones, then the presence of a bond-bond transition would lead to saturation of the available oscillator strength, which would lead to suppression of the bond-continuum transition, which should be studied in this test.
To check this important parameter, two samples HM4229 and HM4230 were fabricated, differing in the width of the quantum well and doping. Sample HM4229 contained GaAs quantum wells 4 nm thick (with a width L QW = 4 nm), each of which was doped with a density of 5 × 10 12 cm -2 . And the HM4230 sample contained quantum wells (L QW= 3.5 nm) doped at 4.77 x 10 12 cm -2 .

Image # 3: coupling-continuum optical transition in pure QW without a surrounding photonic resonator. 3 - measurement of transmission at 300 K for samples with QW of different width L QW ; 3b - 3e are bond-bond ( 3b and 3c ) and bond-continuum transitions ( 3d and 3e ) schemes in doped quantum wells.
-* — , , , () , .Diagrams 3b - 3f show that transitions of different types (bond-bond and bond-continuum) in different single-particle states of the QW potential undergo opposite frequency shifts with decreasing L QW : the former have a blue shift * , the latter have a redshift * .
- * ( -) — (, ).
(Infrared absorption of multiple quantum wells: bound to continuum transitions)
Blue shift * - a phenomenon when the wavelength of radiation decreases and the frequency increases.This made it possible to evaluate the nature of the optical transition by analyzing the transmission spectrum of two samples before using gold ( 3a ).
Redshift * - a phenomenon where the wavelength of radiation increases (light becomes redder, for example), and the frequency and energy decrease.
There is a very wide absorption here, which (being transverse magnetic polarization) is associated with doped quantum wells. A narrower region of about 140 meV is also observed, which is the edge of the continuum. Scientists note that this function does not lead to a blue shift with decreasing L QW , but shows the transfer of spectral weight to the red part of the spectrum. The bond-bond transition would then lead to a blue shift of the order of tens of millielectronvolts, proving the continuum-bound nature of transitions in pure QWs.
As mentioned earlier, all samples were fabricated within a metal-semiconductor-metal lattice and metal pins of width p ( 2a and 2b ). Since the electromagnetic field is extremely localized under the metal fingers, the system essentially behaves like a Fabry – Perot resonator * .
Fabry – Perot resonator * is an optical resonator in which parallel mirrors are directed towards each other. A resonant standing optical wave can form between these mirrors.Several devices were manufactured based on gratings with an area of 200 x 200 μm with a step in the range from 800 nm to 5 μm, which allows covering a wide frequency range ( 2b ). Reflectance data were obtained for each device at 78 K using a FTIR spectroscope equipped with a very compact cryostat ( 2c ).

Image # 4: Experimental reflectivity data. 4 - data on the reflectivity of the doped sample HM4229 depending on the resonator frequency; 4b - reflectance data for HM4229 (red) and a pure resonator (green) for frequencies ω = 157.8 meV (solid lines), ℏω = 147 meV (dashed lines) and ℏω c = 141.5 meV (dash- dotted lines); 4c - line width for various vibrations as a function of vibrational energy.
The results of this analysis are presented in the graphs above. Figure 4a shows a map of the reflectance of sample HM4229 at 78 K as a function of the frequency of the pure resonator. If an absorption continuum is observed above the ionization threshold (shown by the black horizontal dotted line), then a narrow polariton resonance appears below. It is redshifted by more than 20 meV with respect to a pure resonator.
Peak frequencies were plotted on a color map using multiple Lorentz data fit. Red triangles and blue squares represent frequencies below and above the identified ionization threshold, respectively. For comparison, green circles mark the frequency of a pure resonator measured on an undoped sample.
Below the ionization threshold, the lifetime of a discrete polariton mode is mainly limited by the lifetime of the cavity. Above, there is a spectrum of communication-continuum, in which only very extended and vague features can be identified.
Comparison of the spectra of doped and undoped samples showed that a discrete resonance appears in the doped sample below the edge of the continuum, whereas in an identical but electromagnetically unbound sample it is absent.
Such a hybrid discrete state can be described as a polariton, the electron density of which relative to the ground state is:
∆N (z) = P [| Ψ e (z) | 2 - | Ψ g (z) | 2 ]where P (in the range 0 ... 1) is the weight of the polariton component of matter; Ψ g (z) is the normalized wave function of an electron in its ground state; Ψ e (z) is the wave function of a localized electronic state generated by the interaction of light and matter.

Image No. 5: calculations P. 5 - eigenmodes obtained using a theoretical model with parameters selected to match the experimental reflectance data on the color map; 5b are parameters extracted from 5a that are used to calculate P for the discrete polariton mode.
At 5athe result of using the theoretical model for modeling the observed reflectance spectrum and comparing it with experimental data is visually displayed. These parameters allowed the calculation of P ( 5b ).
It follows from this model that the discrete resonance below the ionization threshold is clearly defined for nonzero values of P, demonstrating a significant filling of the electron wave function Ψ e (z) generated by the light .
For a more detailed acquaintance with the nuances of the study, I recommend that you look into the report of scientists and additional materials to it.
Epilogue
This experiment made it possible to demonstrate the possibility of coupling an ionizing transition with a photonic resonator, which leads to a nonperturbative modification of the electronic structure of the system.
The result is a hybrid polariton excitation, the material component of which is a bound state generated by the interaction of light and matter, consisting of an electron and a hole, held together due to their interaction with a transverse electromagnetic field.
According to scientists, the ability to tune the properties of a material due to the connection with the photon field of a microcavity is an extremely promising direction.
In this work, they were able to create a device, limited on two sides by golden mirrors, which captured photons and focused light energy onto electrons, which dramatically strengthened the connection between light and matter. In the course of experiments, it was noticed that a negatively charged electron ejected by a photon remains trapped in a quantum well, associated with other negatively charged electrons. Moreover, this configuration remains stable due to the action of photons.
In other words, this study shows the possibility of creating a new type of artificial atoms, the electronic configurations of which can be customized at will.
Photonics is a fairly young branch of science, but at the same time its influence is growing every year, due to this kind of research. Light, like many other phenomena, can be compared with Schrödinger's cat: on the one hand, everything is clear and obvious, but if you dig deeper, a simple truth becomes obvious - no matter how many answers a person receives, there will always be more questions. Nevertheless, in the search for answers to questions, at least in science, it is not so much the answer itself that is important as the path leading to it.
Thanks for your attention, stay curious and have a good work week, guys. :)
A bit of advertising
Thank you for staying with us. Do you like our articles? Want to see more interesting content? Support us by placing an order or recommending to friends, cloud VPS for developers from $ 4.99 , a unique analogue of entry-level servers that we have invented for you: The Whole Truth About VPS (KVM) E5-2697 v3 (6 Cores) 10GB DDR4 480GB SSD 1Gbps from $ 19 or how to divide the server correctly? (options available with RAID1 and RAID10, up to 24 cores and up to 40GB DDR4).
Is Dell R730xd 2x cheaper in Equinix Tier IV data center in Amsterdam? Only we have 2 x Intel TetraDeca-Core Xeon 2x E5-2697v3 2.6GHz 14C 64GB DDR4 4x960GB SSD 1Gbps 100 TV from $ 199 in the Netherlands!Dell R420 - 2x E5-2430 2.2Ghz 6C 128GB DDR3 2x960GB SSD 1Gbps 100TB - From $ 99! Read about How to build the infrastructure of bldg. class with Dell R730xd E5-2650 v4 servers at a cost of 9000 euros for a penny?