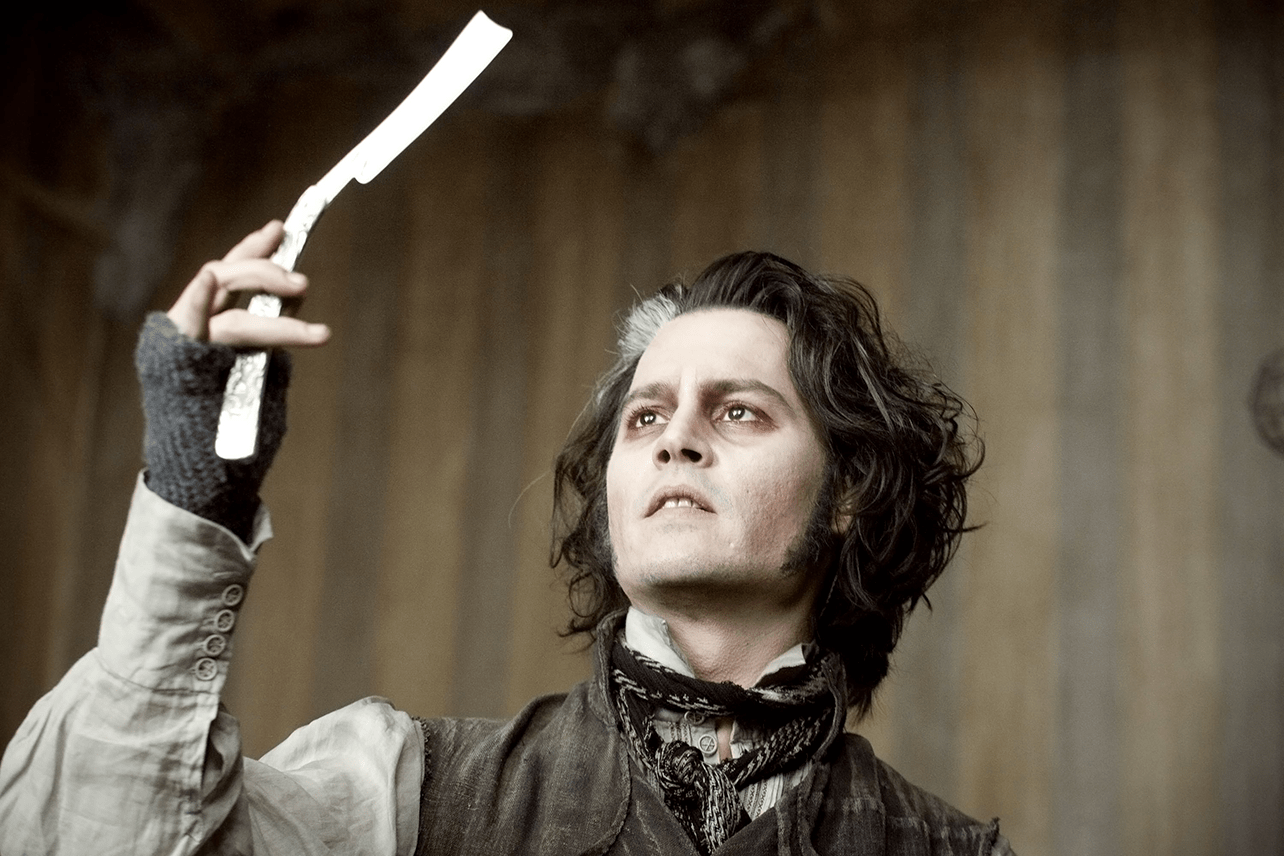
Have you ever wondered how certain objects that surround us every day work. How the refrigerator cools food, how the microwave returns them to their former warmth, how does Wi-Fi work, why the windows do not let rain drops, etc. To some, such questions may seem a little childish, naive and even slightly useless. It works and that's it, but how it doesn't matter anymore. Nevertheless, scientists from MIT (Massachusetts Institute of Technology, USA) decided to answer one of these questions, namely - why do steel razors dull after shaving? What mechanical processes occur during shaving, how human hair, being 50 times softer than steel, damages it, and what is the practical application of this research? We will find answers to these unusual questions in the report of scientists. Go.
Research basis
Over the long years of evolution and development of the social component of our species, we have managed to create a great variety of sharp objects from a variety of materials. Unfortunately, the purpose of most of them is to inflict damage on the enemy: swords, daggers, arrowheads, etc. But there are also quite a few pacifist "wits": razors, scissors, knives, sickles, scythes, etc.
Over the centuries, people have created new alloys and techniques aimed at increasing the degree and prolonging the longevity of the sharpness of the objects described above. The very process of cutting something is present in many industries: medicine, household appliances, food industry, etc. Each of the cases has its own characteristics, but the principle, therefore, and the requirements for the blades remain the same - sharpness and hardness (durability).
For example, a typical metal material used for razor blades is high carbide martensitic * stainless steel plate honed to a 17 ° wedge geometry with a 40 nm radius to obtain the desired sharpness ( 1A ).
Martensite * is the main structural component of hardened steel, which is an ordered supersaturated solid solution of carbon in α-iron with the same concentration as the initial austenite (high-temperature face-centered modification of iron and its alloys). Martensite is a microstructure of acicular (lamellar) and lath (packet) type.

Image # 1
On top of this material is often used even harder - diamond-like carbon, and on top of the latter a layer of polytetrafluoroethylene is applied to reduce friction.
Despite such a complex structure, razors still become dull over time, in contact with a material ~ 50 times softer - hair (hardness scale by 1A ). Razors are not unique in this issue, as other applications for blades of one nature or another also experience a decrease in sharpness over time. For example, a kitchen knife becomes dull even when used exclusively for slicing cheese or potatoes.
However, there is a big difference between kitchen knives and razors. Yes, bluntness occurs both there and there, but in razors the blunting mechanism itself is much more complicated.
First, the two interacting materials have hierarchical microstructures with anisotropic and size-dependent mechanical characteristics. The lath martensitic steels have a hierarchy of primary austenite, packets, blocks, subblocks and lath boundaries, as well as a high density of inhomogeneously distributed dislocations. Intermediate carbon is trapped in the solid solution during quenching, but tempering or automatic tempering * can cause carbon to coat dislocations or precipitate as carbides
Tempering * - the process of heat treatment of an alloy or metal hardened to martensite, the main elements of which are the decomposition of martensite, polygonization and recrystallization.All these structural features provide martensite with high hardness, but non-uniform micromechanical response.

Hair in a section.
Likewise, human hair is a highly anisotropic composite with a non-circular cross-section and an average diameter of 80 to 200 microns. The outer layer of the hair is a hard cuticle (~ 170 MPa), which forms a sheath of cells arranged like roof tiles. The middle layer (cortex) is three times softer and consists of a hierarchy of fibrils extended along the direction of the hair. In the very center of the hair there is a medula - a hollow inner layer that has a fairly small mechanical contribution to the cutting ability of a razor. Since hair is hygroscopic, in the presence of moisture, its cellular structure changes to accommodate water molecules, reducing both the modulus of elasticity and the yield point.
Both the blade and hair are anisotropic and exhibit different mechanical properties depending on size. This produces a mechanical response that is dependent on the stress state and on the volume that contributes to the deformation.
Another important factor is the fact that the boundary conditions for joint deformation of the hair and the razor blade can literally change during one shaving operation ( 1B ).
As the scientists explain, during shaving, each individual hair can be represented as a flexible cantilever, quasi-fixed at one end towards the skin and completely free at the other. In this configuration, the hair can bend freely as the blade approaches and penetrates during cutting, affecting the deformation mode.
Hair predominantly undergoes the fracture mechanics of a type I solid (opening - tensile stress in relation to the crack plane). Depending on whether the hair bends or not, stress is applied either to both surfaces of the crack (straight indentation, g = 0 °), or only to one of the two surfaces. This leads to the appearance of pure destruction of type II in the first case, or to mixed destruction of type II and III in the second case.
Fracture mechanics of solids are divided into three main types according to the method of application of the force that promotes crack propagation:
- Type I (opening) - tensile stress in relation to the crack plane;
- Type II (slip) - shear stress acting parallel to the crack plane and perpendicular to the crack front;
- III () — , .
As we can see, there are many complex aspects behind conventional shaving that require detailed consideration for better understanding, as scientists themselves claim.
For this, they conducted this study, in which the object of study was blades made of martensitic stainless steel. Scanning electron microscope (SEM / SEM) and electron backscattering diffraction (EBSD) analysis revealed their lath martensite matrix ( 1A ) with non-uniformly distributed carbides (inset at 1A ). We also measured hardness values within 70 μm from the tip (the average value was 8.7 ± 0.7 GPa). The variability of this indicator is caused by the presence of carbides, retained austenite, and heterogeneity of the martensite substructure.
To measure the evolution of blade wear in real-life shaving conditions, step-by-step trials of disposable razor cartridges (sample 1 in the image below) were performed, tracking different areas with an SEM after different stages of use (video # 1).
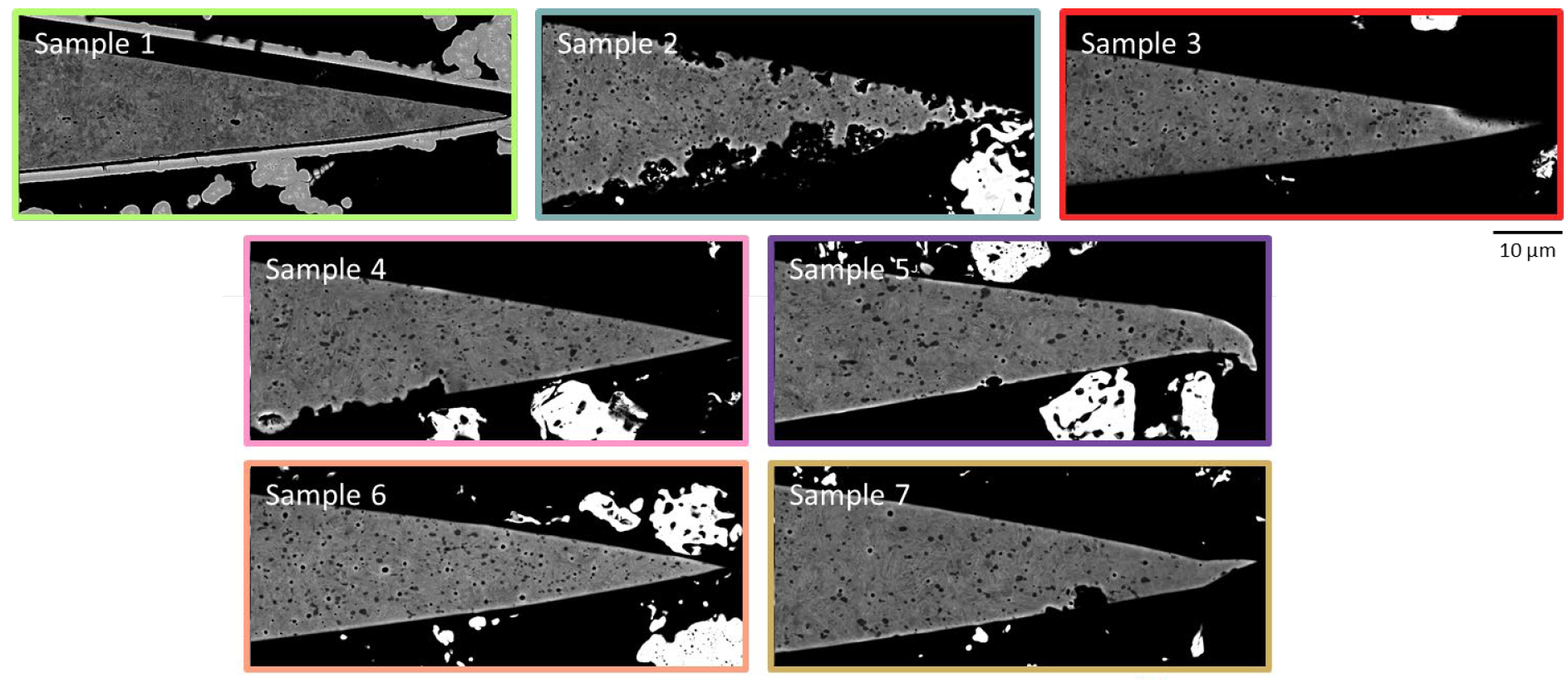
Phased wear tests (after 1, 5 and 10 shaves).
Next, a quantitative determination of the average rate of blade wear along the sharp edge was carried out using image analysis. The wear rate was low: 12 Nm 3 / Nm after 5 shaves and 13 Nm 3 / Nm after 10 shaves. However, these tests revealed the appearance of chips along the sharp edge ( 1C ). Although razor blades are sharp and flat at the macro level, they are "rougher" at the micro scale, even when unused ( 1C-1 ). It is from these irregularities during shaving that microcracks originate ( 1C-2 ). Of all the protrusions, while it may not even be the largest of them, only a small part of them forms cracks.
These microcracks initially propagate perpendicular to the edge ( 1C-2 ) and then deflect in their direction, forming the final cleavage geometry ( 1C-3 ).
Deformation traces ( 1C-2 ) show that the part of the sharp edge belonging to the created cleavage bends out of plane when the direction of the microcrack changes. The presence of pits in the resulting fracture surface indicates that the martensite structure exhibits plastic deformation prior to fracture. In contrast, hard coatings exhibited brittle fracture features as a result of substrate bending.
To better understand the development of this process before activating other types of fracture (fatigue, corrosion and wear), 25 SEM tests were performed in the microdeformation stage using clips that can hold a blade on one side and single or several hairs on the other (image # 2 and video number 2).

Image No. 2
Experiment on shaving individual hairs using SEM.
Making the blade more realistic by tilting the blade 21 ° to the shaving direction ( 2B ) resulted in an unequal force component and in turn plastic deformation and chipping in several cases ( 2C ).
Tests using hairs of different diameters allowed us to conclude that the size of the cleavage does not depend on the diameter of the hair, nor on the number of successively cut hairs, nor on the angle of cut.
The same part of the sharp edge can cut multiple hairs at different angles g ( 1B ) without causing visible deformation until the blade suddenly begins to break (video below).
Experiment on shaving multiple hairs using SEM.
It has also been found that chipping occurs most often at the edges of the hair. For example, a single hair can create two splits on a blade, each of which starts at one end of the hair ( 2C ).
In addition, chips usually extend beyond the highly deformed ~ 5 μm region near the tip of the blade ( 1C ). Therefore, the observed phenomenon is not related to the effects caused by honing.
Comparison of SEM images from two variants of experiments (step-by-step with moisturizing the hair and permanent with dry hairs) shows an identical mechanism of destruction in both cases (except for an increase in the intensity of destruction in the first case).
Further, three-dimensional modeling by the finite element method was used to determine the role of irregularities and the direction of the applied load on deformation and chipping ( 3A - 3C ).

Image # 3
Martensitic steel was modeled as a homogeneous isotropic elastoplastic material with a yield strength of ~ 1690 MPa. Depressions (slots / notches / notches) with a size determined by SEM analysis (for example, 1C-1 ) were added to it . This made it possible to reproduce the extreme state of unevenness along the sharp edge, and to simulate the cutting stress on the blade as a uniformly distributed surface adhesion (50 MPa) acting only on one side ( 3A ).
The first simulation aimed to locate the groove with the highest stress intensity versus the direction of the applied stress.
As seen in 3B , direct indentation of the hair (0 ° surface tension) causes an increase in stresses at the indentations in contact with the hair, but these values are not high enough to promote plastic deformation. In contrast, shaving hair at an angle creates higher stress values in the region of the blade in contact with the hair, with maximum stress in the groove that contacts the edge of the hair.
Analysis of stresses as a function of the angle of adhesion to the surface ( 3C ) showed:
- , ( 8.5°);
- , , ;
- , , ;
- , .
These simulation results are in complete agreement with the experiments, confirming their accuracy. However, there were still discrepancies: microcracks and chips in the experiments were observed at a lower angle than in the simulation.
To clarify this difference, scientists hypothesized a process that links the heterogeneity of the lath martensitic structure of the blade with an increase in sensitivity to microcracks ( 3D - 3H ). The model was adapted to calculate the energy release rate of an interlayer lateral crack in a thin bimaterial semi-infinite plate of constant thickness for mixed fracture (type II + type III), with the crack potentially propagating along the interface between the two materials ( 3D). Further, a stress of 50 MPa was applied, in parallel changing the direction of the load between pure mode II and pure mode III. In this case, materials were considered with the same Poisson's ratio (0.3), but different Young's moduli.
As a result, it was found that the release of energy increases with the transition from mode II to mode III. Changing the Young's moduli of two materials while maintaining their mean value constants causes a vertical shift in the energy release rate curve. This suggests that for two dissimilar materials, the probability of crack propagation will be much higher than for a homogeneous material with similar properties.
Next, the scientists conducted another series of three-dimensional parametric modeling to analyze the rate of energy release at the top of a single recess in the blade, taking into account the geometry of the blade (an increase in thickness in front of the recess itself) and with different directions of crack propagation ( 3E - 3H ). One or two different materials were used on opposite sides of the groove.
Measurement of the force required to cut a single hair.
The energy release rate for a crack propagating along its original direction (q = 0 °) increases as the stress component of mode III ( 3F ) increases . The rate of energy release also depends on the direction of crack propagation ( 3G ). The critical direction corresponding to the maximum rate of energy release depends both on the direction of the load and on the combination of materials ( 3H ).
When a load is applied to a soft material adjacent to a hard material (C and S in the diagram, respectively) with an interlayer depression, the crack is more likely to propagate than in the opposite configuration. The critical angle of crack propagation in this situation will also be smaller ( 3G ).
In addition, microcracks originating on a sharp edge propagate at an angle relative to the blade axis (z-axis at 3E ), deviating towards the load application region.
This propagation path is determined by the asymmetric component of the force, which contributes to the bending of the crack from its original plane, and the geometry of the blade, the thickness of which increases along the axis of the blade.
Distribution of stresses in the blade at different shaving angles.
If we assume that the surface energy of the material is constant, then the energy losses due to crack propagation along this axis will be greater than when the crack propagates in the direction of constant (or less increasing) thickness. If propagation continues along this axis, it will lead to a large area of propagation per unit length. For the same reason, the crack eventually turns back towards the sharp edge of the blade, forming a chip.
To confirm the mechanistic effects of microstructural heterogeneity predicted by analytical and numerical results, hair shaving experiments were performed. In these experiments, a scanning microscope and a focused ion beam allowed a detailed view of the microstructure during deformation. The blades used in the experiment were partially milled, thereby creating a contrast between the martensite matrix and carbides, allowing to reveal the mechanisms of damage (pictures below).

Microscopy of a semi-milled blade.
Experiments have shown that microcracks usually originated at the interfaces between martensite and carbide, which border on irregularities (left to B) and when the hair is in contact with the more pliable component of the blade. The microcracks then propagate at an angle, causing decohesion at several carbide-matrix interfaces or cracking of the carbide (right to B), as well as microplasticity in the martensitic regions between them.
The totality of the results of modeling, calculations and experiments suggests that shaving can cause the initiation of damage, their growth and coalescence * (in the form of chips) in lath martensitic steel. It also became clear that chips appear earlier than other types of damage.
Coalescence * - fusion of particles on the surface of a body (in this case) or inside a moving medium (gas, liquid).
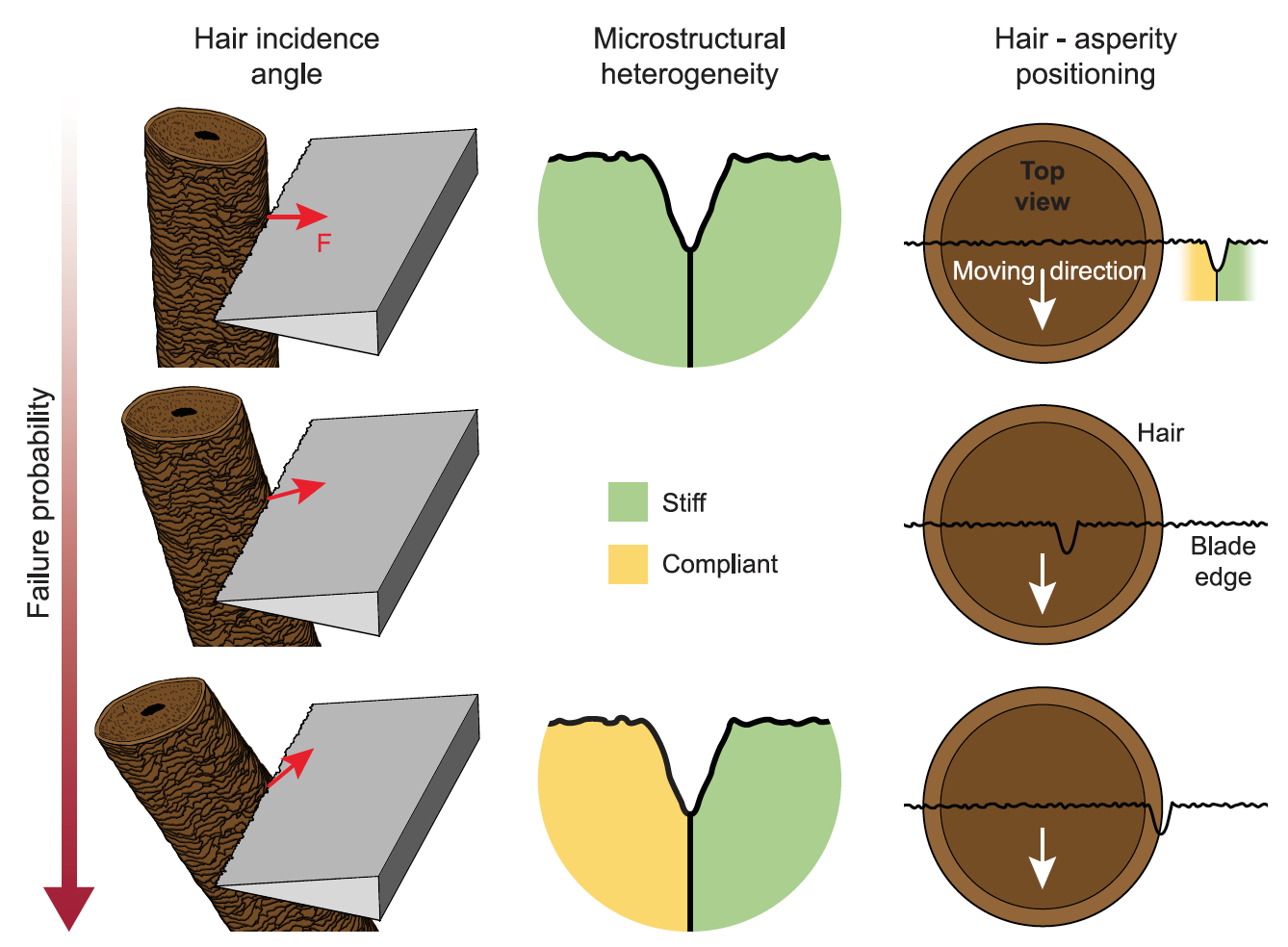
Image # 4
This process requires a combination of several factors for its implementation (image above):
- a sufficient level of bending of the hair to create stresses with a significant component of the III-type;
- caused by processing unevenness on the edge of the blade with microstructural components with rather different properties on each side;
- the hair is positioned so that the extreme point is aligned with the above unevenness (to ensure maximum stress amplification) and is in contact with the side containing the more compliant component.
If we take into account that such conditions are extremely rare at the same time, it becomes clear why conventional razors become unsuitable for shaving not immediately (after the first use), but after a certain number of shaving cycles.
For a more detailed acquaintance with the nuances of the study, I recommend that you look into the report of scientists and additional materials to it.
Epilogue
The fact that razors become dull after a certain number of shaves is well known and understood. However, in this work, scientists decided to consider in detail the smallest processes that occur during the wear of the razor blades. This work is not so much about razors as about alloys and other materials used in various industries to cut something. For knowing what causes damage to the material, you can find a way to avoid it.
The scientists noted that blades are more prone to chipping if the microstructure of the steel is not uniform. Naturally, the angle at which the blades contact the hairs, as well as defects in the microstructure of the blades, also play an important role in the formation of cracks.
Curiously, the actual wear on the steel razor blades did not increase much during the experiments. The blades remained sharp for a long time, but chips formed on their edges, which disrupt the "performance" of the razors. In this case, chips were formed only in certain places, i.e. under certain conditions: when the blade was in contact with the hair at an angle, when the steel of the blade was uneven in composition, and when the hair was in contact with the blade in a place where its unevenness was higher.
The main conclusion is quite simple - razors become dull due to the heterogeneity of their composition. Hair is much softer than steel, but the steel used in the blades is a kind of composite material, which greatly reduces its strength and resistance to damage. If you reduce the heterogeneity of the material, then you can significantly improve its mechanical characteristics.
In the future, scientists intend to continue their research, as well as start a new one, in which they will look for new "recipes" for steel blades, which will subsequently be much more durable, sharper and stronger than the current ones.
Thanks for your attention, stay curious and have a good work week, guys. :)
A bit of advertising
Thank you for staying with us. Do you like our articles? Want to see more interesting content? Support us by placing an order or recommending to friends, cloud VPS for developers from $ 4.99 , a unique analogue of entry-level servers that we have invented for you: The Whole Truth About VPS (KVM) E5-2697 v3 (6 Cores) 10GB DDR4 480GB SSD 1Gbps from $ 19 or how to divide the server correctly? (options available with RAID1 and RAID10, up to 24 cores and up to 40GB DDR4).
Is Dell R730xd 2x cheaper in Equinix Tier IV data center in Amsterdam? Only we have 2 x Intel TetraDeca-Core Xeon 2x E5-2697v3 2.6GHz 14C 64GB DDR4 4x960GB SSD 1Gbps 100 TV from $ 199 in the Netherlands!Dell R420 - 2x E5-2430 2.2Ghz 6C 128GB DDR3 2x960GB SSD 1Gbps 100TB - From $ 99! Read about How to build the infrastructure of bldg. class with Dell R730xd E5-2650 v4 servers at a cost of 9000 euros for a penny?