
1. Introduction
Quantum optics specialists have long been interested in the human visual system, which is likely sensitive to single photons. Early experiments were limited by the incoherence of classical light sources, but the era of true single-photon sources and tunable photon statistics has opened up new areas of research, including measuring the quantum efficiency of twilight eye photoreceptor rods (about 33%) [1], and measuring photon statistics from various light sources in which rods are used as sensors [2]. A recent experiment has provided better evidence that the visual system can detect one photon [3], while another investigated the temporal summation in the visual system for several photons [4].These advances in single-photon vision research provide a unique opportunity to study quantum effects with the visual system, including superposition and entanglement. This article provides a brief overview of previous research into single-photon vision and current capabilities, and proposes two experiments to study the perception of the superposition state and the use of a human observer as a detector in the Bell test.
2.
Soon after the concept of light as photons emerged in the early twentieth century, it became clear that the statistics of individual photons were likely to be important in determining the lower threshold of human vision [5]. One of the earliest and most famous lower threshold experiments was carried out by Hecht, Schleer and Pirenne in 1942 [6]. In their studies, subjects observed very dim flashes of light with an average number of photons ranging from 50 to 400. After each flash, the subjects (each of the three study co-authors) were asked whether it was visible or not? The average number of photons in flares was varied, and it was determined how often the subjects detected a flare at each level. Assuming that the number of photons detected by the visual system in each test is a random variable obeying the Poisson distribution,and that a certain threshold number of photons n was required for perception, Hecht et al. calculated that the threshold for vision was between 5 and 7 photons, depending on the subject (Fig. 1).
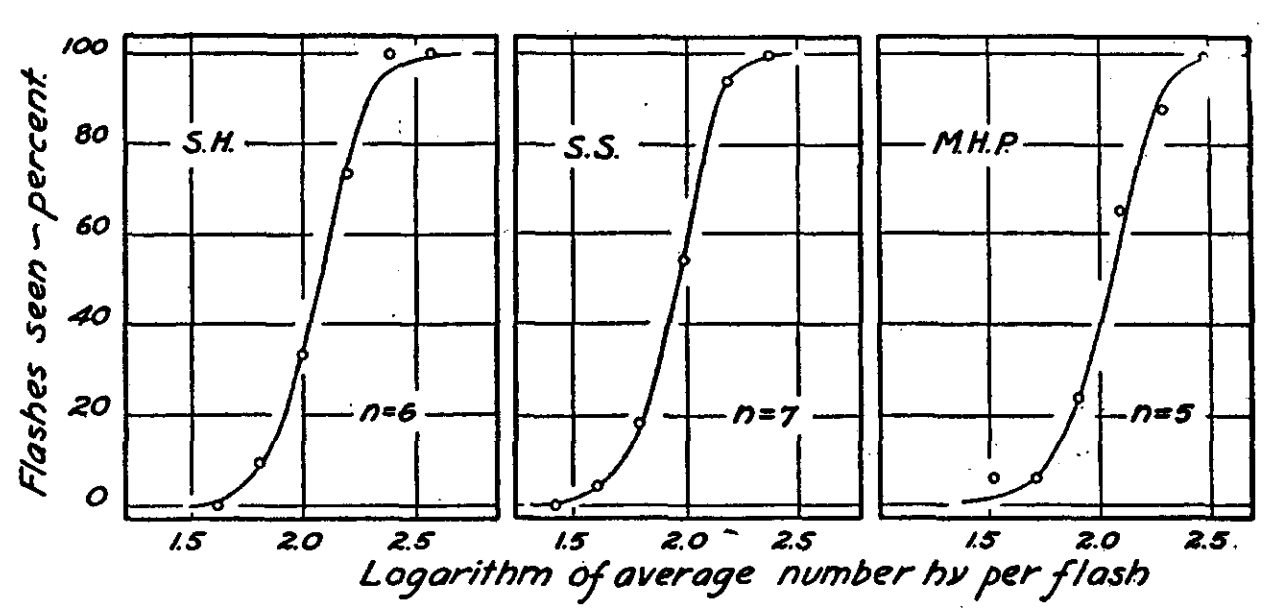
Figure: 1. Data from Hecht et al. [6]. Fitting the Poisson model to measure the relationship between the average number of photons in flares and the frequency with which the subject reported being visible gives an estimate of the visual threshold n.
This experiment was one of the first to provide evidence that rods can respond to single photons: flashes of light fell on an area containing about 500 rods, so if only 5-7 photons were detected, any single rod could not detect more than one. However, there are several problems in this experimental setup that could lead to overestimation of these values. The most important is the request to simply report whether the outbreak was noticed or not, which could lead to an overestimation of the threshold in comparison with the true one, due to possible false-positive responses of the subjects.
Moreover, in later experiments in which subjects were instructed to rate weak flashes of light on a scale from 0 to 6, they found a possible lower threshold for vision of just one photon (for some subjects) [7]. In vitro measurements of individual rod cells have also shown that the cells produce discrete electrical signals in response to dim light bursts with minimal signal levels that appear to correspond to single photons [8] (see Fig. 2).
However, all these experiments were limited by the incoherence of radiation from classical light sources, which cannot produce single photons. The development of single-photon sources has created new opportunities in vision research, which are discussed in Section 3.

. 2. () . , , . () t = 0. ~1, ~500. () , . 1 2 [9].
3.
Single-photon sources have been developed for quantum optics and quantum information research, and include sources based on single atoms [10], nitrogen-substituted vacancies in diamond [11, 12], quantum dots [13], and spontaneous parametric scattering with decreasing frequency ( spontaneous parametric down-conversion - SPDC) [14]. SPDC sources are in many ways ideal for single-photon vision studies, as they can be very bright, can emit light in a wide wavelength range (rods are most sensitive around 500 nm), and have high efficiency, mainly limited by optical losses. With some modification, they can also easily produce polarization-entangled pairs of photons, and other degrees of freedom [15].
In fig. 3 shows an example of an SPDC steam source developed in our laboratory and optimized for human vision research [16,17]. The heralding efficiency of this source (the probability that a photon is sent to an observer if a messenger photon is detected ) was 38.5%. It generates single photons with a wavelength of 505 nm near the peak of the spectral sensitivity of the rods.

. 3. . (VA). 562 505 (BBO); 562- (SPAD) ( , ) FPGA. 505- 25- , (PBS) (FPC); (PC), PBS. . 505- (HWP) PBS, (. 4). 505 , .
To study human vision, an observation station is also required, with the help of which photons are delivered to the subject's eyes (Fig. 4). Our station allows you to deliver photons to one or two spatially separated points of the retina, at an angle of approximately ± 16 degrees to the left and right of the fovea- central fossa. This feature allows for improved experimental design compared to Hecht et al .: instead of asking subjects whether they saw a photon or not, one can randomly (using PC and PBS as shown in Fig. 3) send a photon to the left or right point and ask where he was seen. This removes the artificial threshold effect that can occur when the subject is asked to rate the presence or absence of a stimulus. If the subjects choose "left" or "right" with an accuracy significantly greater than 50%, then we can conclude that they saw the stimulus. The disadvantage of this approach is that due to the relatively high optical loss in the eyes (estimated ~ 90-97%), a large number of long series of tests are usually required to demonstrate the effect,since in most of them the subjects do not actually detect the photon.
We used this source to study how the visual system summarizes photons within a short time window [4], others used a similar SPDC source and a different experimental setup (replacing left and right with earlier and later photon delivery times) to show that test subjects can achieve an accuracy of over 50% for single photons. Subsequent research with a much larger number of experimental trials and improved experimental design (including an equal number of control trials in which no photons are present) will be essential to confirm this result. However, we believe that humans are indeed capable of detecting single photons. An exciting opportunity isthat a similar single-photon source can now be used to study quantum effects using the visual system. The two proposed experiments are presented in Section 4.
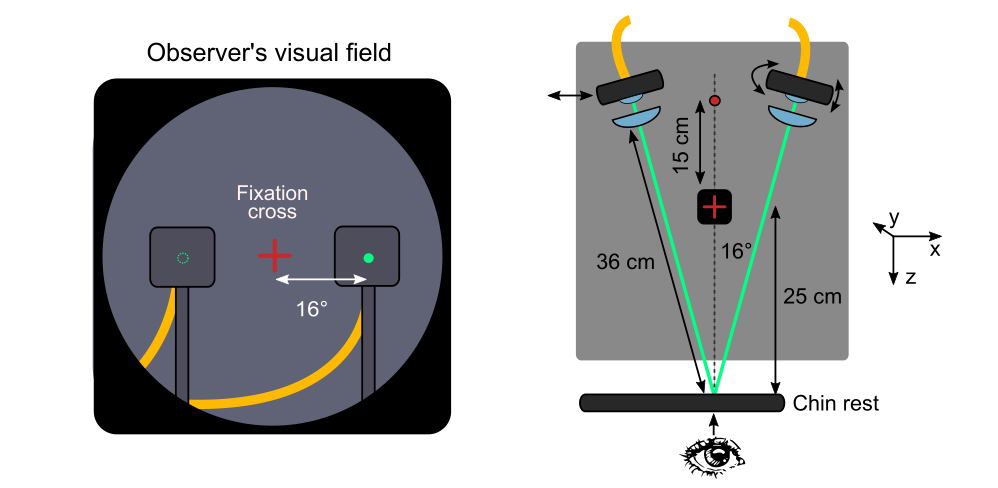
Figure 4. Subject's field of view and schematic top view of the observation station. The fixation cross (shown in the field of view not to scale) consists of a faint 700nm LED behind a crosshair mask (rods are insensitive to far red wavelengths). The left and right beams are aligned with the subject's right eye when the subject is fixed on the chin rest.
4. Suggested experiments: superposition and entanglement
If a person can detect single photons, then a wide range of exciting works and experiments opens before us in the future. By examining how subjects directly interact with and measure quantum phenomena, we can test the predictions of standard quantum mechanics and even give the human observer a direct role in the local realism test.
4.1
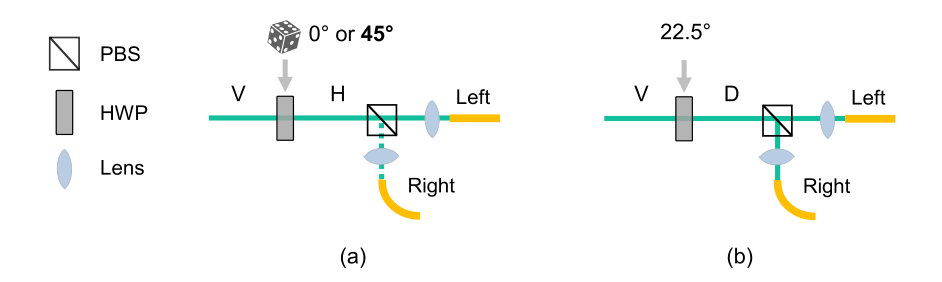
. 5. (a) /, . (HWP) 0°, 45°, , . . (b) . HWP 22.5°, (V) (D), V. (PBS) , . , , () , .
One relatively simple test we can perform is to determine if humans perceive any difference between a photon in a superposition state and a classical mixed state. The superposition experiment in the visual system has been of great interest for many years, and several approaches have been proposed [18,19]. To perform this experiment, you can use the setup shown in Fig. 3, but in addition to tests in which one photon is present on either the left or right side of the retina, tests can also be performed with a photon randomly represented in a superposition of the left and right sides. This is easily achieved by rotating the half-wave plate shown in Fig. 5 to the 22.5 ° position, to obtain the state
As in the single-photon vision test, the subject is asked to report from which side the flash was visible in each of the tests. According to standard quantum mechanics, there should be no difference in perception between the same superposition and the same classical mixture. Any statistically significant difference in the ratio of left and right responses between these two conditions (after carefully considering any deviation in the hardware) will indicate an unexpected effect, and may have implications for alternative interpretations of quantum mechanics (eg macrorealism [20,21]).
4.2 Bell test with human observer

Figure: 6. Simplified scheme of Bell's test with replacement of one detector by a human observer. When one of the detectors on side A indicates that the photon has been measured with the, , . - : (PC) , (HWP) . PC , HWP ( ). (LC) .
Another exciting experiment we can do is test local realism with a human observer as a detector. The first step would be to replace one detector with a human observer and the other with measurements with our highly efficient photon counter (see Fig. 6). By simultaneously pumping two orthogonal nonlinear crystals, one can obtain polarization-entangled pairs of photons [15, 22, 23]. Then one can use the well-known CH ( Clauser-Horne ) inequality [24], which connects joint and single probabilities for settings on polarizing analyzers A and B:
It can be shown that any theory supporting local realism must obey this inequality. In the initial experiment, we use single-photon detectors to measure all terms except... When using the optimal set of settings for analysis, the inequality simplifies to
A forced selection design, similar to the single-photon vision test, can be used to control the low likelihood that the subject will detect a photon in any of the tests. If the measurement on side B indicates the desired result for the term
5. Conclusion
Now that experiments with single-photon sources have shown that humans can probably detect single photons, a wide range of interesting new experiments can be proposed, both in physics and psychology. This article reviewed previous research on the lower limit of human vision and presented two possible experiments to test quantum mechanics using the visual system, including states of superposition and entanglement. The main problem for these and other single-photon vision experiments will be the low probability that a photon will be transmitted to photoreceptors and detected in separate tests (perhaps 5-10%, provided an absolutely effective source), and, accordingly, the requirements for very large series of tests.
Despite the fact that the presence of a person as an observer makes the proposed experiments unique and interesting, we emphasize that they are not supposed to test the influence of the observer's consciousness on the results of these experiments; rather, these experiments use the unique capabilities of the visual system to test the predictions of quantum mechanics, and may even clarify experimental limitations on alternative propositions such as macrorealism.
There is also a number of interesting psychophysical studies that our single photon source can use. Other aspects of time stacking at the lowest light levels can be investigated, such as whether low light is perceived to be quantized. Using deformable mirrors and spatial light modulators, spatial stacking can be studied by varying the size of the low-photon stimulus on the retina. More advanced sources that can generate states with a higher number of photons [25] can also be used to measure the visual sensitivity function for a precisely specified number of photons.
BIBLIOGRAPHY
[1] Phan, N. M., Cheng, M. F., Bessarab, D. A., and Krivitsky, L. A., “Interaction of Fixed Number of Photons with Retinal Rod Cells,” Physical Review Letters 112, 213601 (may 2014). [2] Sim, N., Cheng, M. F., Bessarab, D. A., Jones, C. M., and Krivitsky, L. A., “Measurement of Photon Statistics with Live Photoreceptor Cells,” Physical Review Letters 109, 113601 (sep 2012).
[3] Tinsley, J. N., Molodtsov, M. I., Prevedel, R., Wartmann, D., Espigul´ e-Pons, J., Lauwers, M., and Vaziri, A., “Direct detection of a single photon by humans,” Nature Communications 7, 12172 (jul 2016).
[4] Holmes, R., Victora, M., Wang, R. F., and Kwiat, P. G., “Measuring temporal summation in visual detection with a single-photon source,” Vision Research 140, 33–43 (2017).
[5] Bouman, M. A., “History and Present Status of Quantum Theory in Vision,” in [Sensory Communication], 376–401, The MIT Press (sep 2012).
[6] Hecht, S., “ENERGY, QUANTA, AND VISION,” The Journal of General Physiology 25, 819–840 (jul 1942).
[7] Sakitt, B., “Counting every quantum,” The Journal of Physiology 223(1), 131–150 (1972).
[8] Rieke, F. and Baylor, D. A., “Single-photon detection by rod cells of the retina,” Reviews of Modern Physics 70(3), 1027–1036 (1998).
[9] Baylor, D. A., Nunn, B. J., and Schnapf, J. L., “The photocurrent, noise and spectral sensitivity of rods of the monkey Macaca fascicularis,” The Journal of Physiology 357, 575–607 (dec 1984).
[10] McKeever, J., Boca, A., Boozer, A. D., Miller, R., Buck, J. R., Kuzmich, A., and Kimble, H. J., “Deter-ministic Generation of Single Photons from One Atom Trapped in a Cavity,” Science 303(March), 1992 (2004).
[11] Kurtsiefer, C., Mayer, S., Zarda, P., and Weinfurter, H., “Stable solid-state source of single photons,” Physical Review Letters 85(2), 290–293 (2000).
[12] Beveratos, A., Brouri, R., Gacoin, T., Poizat, J.-P., and Grangier, P., “Nonclassical radiation from diamond nanocrystals,” Physical Review A 64, 061802 (nov 2001).
[13] Michler, P., Kiraz, A., Becher, C., Schoenfeld, W. V., Petroff, P. M., Zhang, L., Hu, E., and Imamoglu, A., “A quantum dot single-photon turnstile device,” Science 290, 2282–5 (dec 2000).
[14] Hong, C. K. and Mandel, L., “Experimental realization of a localized one-photon state,” Physical Review Letters 56(1), 58–60 (1986).
[15] Kwiat, P. G., Waks, E., White, A. G., Appelbaum, I., and Eberhard, P. H., “Ultra-bright source of polarization-entangled photons,” Physical Review A 60(2), R773® (1999).
[16] Holmes, R., Christensen, B. G., Street, W., Wang, R. F., and Kwiat, P. G., “Determining the Lower Limit of Human Vision Using a Single Photon Source,” in [Conference on Lasers and Electro-Optics 2012], QTu1E.8, Optical Society of America (2012).
[17] Holmes, R., Christensen, B. G., Wang, R. F., and Kwiat, P. G., “Testing the Limits of Human Vision with Single Photons,” in [Frontiers in Optics 2015], FTu5B.5, Optical Society of America (2015).
[18] Ghirardi, G., “Quantum superpositions and definite perceptions: envisaging new feasible experimental tests,” Physics Letters A 262, 1–14 (oct 1999).
[19] Thaheld, F. H., “Can we determine if the linear nature of quantum mechanics is violated by the perceptual process?,” BioSystems 71, 305–309 (oct 2003).
[20] Ghirardi, G. C., Rimini, A., and Weber, T., “Unified dynamics for microscopic and macroscopic systems,”
Physical Review D 34, 470–491 (jul 1986).
[21] Leggett, A. J., “Testing the limits of quantum mechanics: motivation, state of play, prospects,” Journal of Physics: Condensed Matter 14(15), R415–R451 (2002).
[22] Christensen, B. G., McCusker, K. T., Altepeter, J. B., Calkins, B., Gerrits, T., Lita, A. E., Miller, A., Shalm, L. K., Zhang, Y., Nam, S. W., Brunner, N., Lim, C. C. W., Gisin, N., and Kwiat, P. G., “Detection-Loophole-Free Test of Quantum Nonlocality, and Applications,” Physical Review Letters 111, 130406 (sep 2013).
[23] Akselrod, G. M., Altepeter, J. B., Jeffrey, E. R., and Kwiat, P. G., “Phase-compensated ultra-bright source of entangled photons: erratum,” Optics Express 13, 5260–5261 (apr 2005).
[24] Clauser, J. F. and Horne, M. A., “Experimental consequences of objective local theories,” Physical Review D 10, 526–535 (jul 1974).
[25] McCusker, K. T. and Kwiat, P. G., “Efficient Optical Quantum State Engineering,” Physical Review Letters 103, 163602 (oct 2009).
[3] Tinsley, J. N., Molodtsov, M. I., Prevedel, R., Wartmann, D., Espigul´ e-Pons, J., Lauwers, M., and Vaziri, A., “Direct detection of a single photon by humans,” Nature Communications 7, 12172 (jul 2016).
[4] Holmes, R., Victora, M., Wang, R. F., and Kwiat, P. G., “Measuring temporal summation in visual detection with a single-photon source,” Vision Research 140, 33–43 (2017).
[5] Bouman, M. A., “History and Present Status of Quantum Theory in Vision,” in [Sensory Communication], 376–401, The MIT Press (sep 2012).
[6] Hecht, S., “ENERGY, QUANTA, AND VISION,” The Journal of General Physiology 25, 819–840 (jul 1942).
[7] Sakitt, B., “Counting every quantum,” The Journal of Physiology 223(1), 131–150 (1972).
[8] Rieke, F. and Baylor, D. A., “Single-photon detection by rod cells of the retina,” Reviews of Modern Physics 70(3), 1027–1036 (1998).
[9] Baylor, D. A., Nunn, B. J., and Schnapf, J. L., “The photocurrent, noise and spectral sensitivity of rods of the monkey Macaca fascicularis,” The Journal of Physiology 357, 575–607 (dec 1984).
[10] McKeever, J., Boca, A., Boozer, A. D., Miller, R., Buck, J. R., Kuzmich, A., and Kimble, H. J., “Deter-ministic Generation of Single Photons from One Atom Trapped in a Cavity,” Science 303(March), 1992 (2004).
[11] Kurtsiefer, C., Mayer, S., Zarda, P., and Weinfurter, H., “Stable solid-state source of single photons,” Physical Review Letters 85(2), 290–293 (2000).
[12] Beveratos, A., Brouri, R., Gacoin, T., Poizat, J.-P., and Grangier, P., “Nonclassical radiation from diamond nanocrystals,” Physical Review A 64, 061802 (nov 2001).
[13] Michler, P., Kiraz, A., Becher, C., Schoenfeld, W. V., Petroff, P. M., Zhang, L., Hu, E., and Imamoglu, A., “A quantum dot single-photon turnstile device,” Science 290, 2282–5 (dec 2000).
[14] Hong, C. K. and Mandel, L., “Experimental realization of a localized one-photon state,” Physical Review Letters 56(1), 58–60 (1986).
[15] Kwiat, P. G., Waks, E., White, A. G., Appelbaum, I., and Eberhard, P. H., “Ultra-bright source of polarization-entangled photons,” Physical Review A 60(2), R773® (1999).
[16] Holmes, R., Christensen, B. G., Street, W., Wang, R. F., and Kwiat, P. G., “Determining the Lower Limit of Human Vision Using a Single Photon Source,” in [Conference on Lasers and Electro-Optics 2012], QTu1E.8, Optical Society of America (2012).
[17] Holmes, R., Christensen, B. G., Wang, R. F., and Kwiat, P. G., “Testing the Limits of Human Vision with Single Photons,” in [Frontiers in Optics 2015], FTu5B.5, Optical Society of America (2015).
[18] Ghirardi, G., “Quantum superpositions and definite perceptions: envisaging new feasible experimental tests,” Physics Letters A 262, 1–14 (oct 1999).
[19] Thaheld, F. H., “Can we determine if the linear nature of quantum mechanics is violated by the perceptual process?,” BioSystems 71, 305–309 (oct 2003).
[20] Ghirardi, G. C., Rimini, A., and Weber, T., “Unified dynamics for microscopic and macroscopic systems,”
Physical Review D 34, 470–491 (jul 1986).
[21] Leggett, A. J., “Testing the limits of quantum mechanics: motivation, state of play, prospects,” Journal of Physics: Condensed Matter 14(15), R415–R451 (2002).
[22] Christensen, B. G., McCusker, K. T., Altepeter, J. B., Calkins, B., Gerrits, T., Lita, A. E., Miller, A., Shalm, L. K., Zhang, Y., Nam, S. W., Brunner, N., Lim, C. C. W., Gisin, N., and Kwiat, P. G., “Detection-Loophole-Free Test of Quantum Nonlocality, and Applications,” Physical Review Letters 111, 130406 (sep 2013).
[23] Akselrod, G. M., Altepeter, J. B., Jeffrey, E. R., and Kwiat, P. G., “Phase-compensated ultra-bright source of entangled photons: erratum,” Optics Express 13, 5260–5261 (apr 2005).
[24] Clauser, J. F. and Horne, M. A., “Experimental consequences of objective local theories,” Physical Review D 10, 526–535 (jul 1974).
[25] McCusker, K. T. and Kwiat, P. G., “Efficient Optical Quantum State Engineering,” Physical Review Letters 103, 163602 (oct 2009).
Translation author additions
In some online publications with popular science topics, publications appeared on the topic of the article and ongoing research ( 1 , 2 ). They provide additional considerations related to the planned research.
Quote with translation from 1
— . , , , , , . , , , «» , , «» «». ? « , », — . , , , - . « », — .
, . , , , . ? — ? ? ? ?
. , , «GRW» ( , ). GRW , ; , . , , — . GRW . , , . « » .
, GRW, , , , . , GRW, , . , — — . , , , , , GRW ( GRW (GianCarlo Ghirardi (1998)) — . ).
, - , , , GRW , , , . , , . « . , », — .
. « , ?» — . « — , . - , , ».
, 2016 , , , , . , ? , ? , ? ( ) ( ), , .
, . « , », — .
: . , ? , - .
« , , . , , , », — — « , , ».
, . , , , . ? — ? ? ? ?
. , , «GRW» ( , ). GRW , ; , . , , — . GRW . , , . « » .
, GRW, , , , . , GRW, , . , — — . , , , , , GRW ( GRW (GianCarlo Ghirardi (1998)) — . ).
, - , , , GRW , , , . , , . « . , », — .
. « , ?» — . « — , . - , , ».
, 2016 , , , , . , ? , ? , ? ( ) ( ), , .
, . « , », — .
: . , ? , - .
« , , . , , , », — — « , , ».
Article 2 is almost completely translated on one of the resources , and reveals the name of the ideological inspirer of these studies.
Quote from her
? , . , , . , , — — . , , , , , ( ) . , — . , . , , .
. 2015 , , , . , . 2017 « »: , , ( ) . BIG Bell Test 100 000 - 2016 . - .
. 2015 , , , . , . 2017 « »: , , ( ) . BIG Bell Test 100 000 - 2016 . - .
Translation author comments
Comments may contain, explicitly or implicitly, the opinion of the author on the topics presented.